A Noelin-organized extracellular network of proteins required for constitutive and context-dependent anchoring of AMPA-receptors
- PMID: 37591201
- PMCID: PMC10441612
- DOI: 10.1016/j.neuron.2023.07.013
A Noelin-organized extracellular network of proteins required for constitutive and context-dependent anchoring of AMPA-receptors
Abstract
Information processing and storage in the brain rely on AMPA-receptors (AMPARs) and their context-dependent dynamics in synapses and extra-synaptic sites. We found that distribution and dynamics of AMPARs in the plasma membrane are controlled by Noelins, a three-member family of conserved secreted proteins expressed throughout the brain in a cell-type-specific manner. Noelin tetramers tightly assemble with the extracellular domains of AMPARs and interconnect them in a network-like configuration with a variety of secreted and membrane-anchored proteins including Neurexin1, Neuritin1, and Seizure 6-like. Knock out of Noelins1-3 profoundly reduced AMPARs in synapses onto excitatory and inhibitory (inter)neurons, decreased their density and clustering in dendrites, and abolished activity-dependent synaptic plasticity. Our results uncover an endogenous mechanism for extracellular anchoring of AMPARs and establish Noelin-organized networks as versatile determinants of constitutive and context-dependent neurotransmission.
Keywords: AMPA-receptor; excitatory neurotransmission; extracellular matrix; long-term potentiation; mass spectrometry; protein-protein interaction; proteomics; secreteted proteins; synaptic plasticity.
Copyright © 2023 The Author(s). Published by Elsevier Inc. All rights reserved.
Conflict of interest statement
Declaration of interests The authors declare no competing interests.
Figures
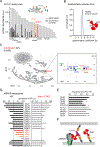
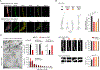
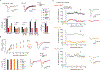
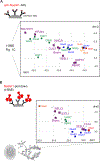
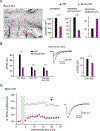
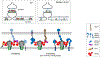
Similar articles
-
Control of Homeostatic Synaptic Plasticity by AKAP-Anchored Kinase and Phosphatase Regulation of Ca2+-Permeable AMPA Receptors.J Neurosci. 2018 Mar 14;38(11):2863-2876. doi: 10.1523/JNEUROSCI.2362-17.2018. Epub 2018 Feb 13. J Neurosci. 2018. PMID: 29440558 Free PMC article.
-
Protein kinase D promotes activity-dependent AMPA receptor endocytosis in hippocampal neurons.Traffic. 2021 Dec;22(12):454-470. doi: 10.1111/tra.12819. Epub 2021 Oct 5. Traffic. 2021. PMID: 34564930
-
AKAP150-anchored calcineurin regulates synaptic plasticity by limiting synaptic incorporation of Ca2+-permeable AMPA receptors.J Neurosci. 2012 Oct 24;32(43):15036-52. doi: 10.1523/JNEUROSCI.3326-12.2012. J Neurosci. 2012. PMID: 23100425 Free PMC article.
-
Regulation of neuronal PKA signaling through AKAP targeting dynamics.Eur J Cell Biol. 2006 Jul;85(7):627-33. doi: 10.1016/j.ejcb.2006.01.010. Epub 2006 Feb 28. Eur J Cell Biol. 2006. PMID: 16504338 Review.
-
Beyond the AMPA receptor: Diverse roles of SynDIG/PRRT brain-specific transmembrane proteins at excitatory synapses.Curr Opin Pharmacol. 2021 Jun;58:76-82. doi: 10.1016/j.coph.2021.03.011. Epub 2021 May 5. Curr Opin Pharmacol. 2021. PMID: 33964729 Free PMC article. Review.
Cited by
-
Cells and Molecules Underpinning Cannabis-Related Variations in Cortical Thickness during Adolescence.J Neurosci. 2024 Oct 9;44(41):e2256232024. doi: 10.1523/JNEUROSCI.2256-23.2024. J Neurosci. 2024. PMID: 39214708
-
Proton-triggered rearrangement of the AMPA receptor N-terminal domains impacts receptor kinetics and synaptic localization.Nat Struct Mol Biol. 2024 Oct;31(10):1601-1613. doi: 10.1038/s41594-024-01369-5. Epub 2024 Aug 13. Nat Struct Mol Biol. 2024. PMID: 39138332 Free PMC article.
-
Activity-dependent diffusion trapping of AMPA receptors as a key step for expression of early LTP.Philos Trans R Soc Lond B Biol Sci. 2024 Jul 29;379(1906):20230220. doi: 10.1098/rstb.2023.0220. Epub 2024 Jun 10. Philos Trans R Soc Lond B Biol Sci. 2024. PMID: 38853553 Free PMC article. Review.
-
Quantitative proteomics of dorsolateral prefrontal cortex reveals an early pattern of synaptic dysmaturation in children with idiopathic autism.Cereb Cortex. 2024 May 2;34(13):161-171. doi: 10.1093/cercor/bhae044. Cereb Cortex. 2024. PMID: 38696595 Free PMC article.
-
Tuning synaptic strength by regulation of AMPA glutamate receptor localization.Bioessays. 2024 Jul;46(7):e2400006. doi: 10.1002/bies.202400006. Epub 2024 May 1. Bioessays. 2024. PMID: 38693811 Free PMC article. Review.
References
Publication types
MeSH terms
Substances
Grants and funding
LinkOut - more resources
Full Text Sources
Molecular Biology Databases