High-Throughput Mass Spectrometry Imaging of Biological Systems: Current Approaches and Future Directions
- PMID: 37206615
- PMCID: PMC10191415
- DOI: 10.1016/j.trac.2023.117055
High-Throughput Mass Spectrometry Imaging of Biological Systems: Current Approaches and Future Directions
Abstract
In the past two decades, the power of mass spectrometry imaging (MSI) for the label free spatial mapping of molecules in biological systems has been substantially enhanced by the development of approaches for imaging with high spatial resolution. With the increase in the spatial resolution, the experimental throughput has become a limiting factor for imaging of large samples with high spatial resolution and 3D imaging of tissues. Several experimental and computational approaches have been recently developed to enhance the throughput of MSI. In this critical review, we provide a succinct summary of the current approaches used to improve the throughput of MSI experiments. These approaches are focused on speeding up sampling, reducing the mass spectrometer acquisition time, and reducing the number of sampling locations. We discuss the rate-determining steps for different MSI methods and future directions in the development of high-throughput MSI techniques.
Keywords: Continuous scanning; MSI; Multimodal; Sparse sampling; Throughput.
Conflict of interest statement
Declaration of interests The authors declare that they have no known competing financial interests or personal relationships that could have appeared to influence the work reported in this paper.
Figures
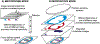
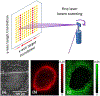
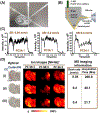
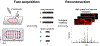
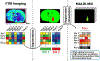
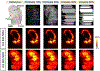
Similar articles
-
High-Throughput Mass Spectrometry Imaging with Dynamic Sparse Sampling.ACS Meas Sci Au. 2022 Oct 19;2(5):466-474. doi: 10.1021/acsmeasuresciau.2c00031. Epub 2022 Aug 15. ACS Meas Sci Au. 2022. PMID: 36281292 Free PMC article.
-
Emerging Computational Methods in Mass Spectrometry Imaging.Adv Sci (Weinh). 2022 Dec;9(34):e2203339. doi: 10.1002/advs.202203339. Epub 2022 Oct 17. Adv Sci (Weinh). 2022. PMID: 36253139 Free PMC article. Review.
-
[Mass spectrometry imaging technology and its application in breast cancer research].Se Pu. 2021 Jun;39(6):578-587. doi: 10.3724/SP.J.1123.2020.10005. Se Pu. 2021. PMID: 34227318 Free PMC article. Review. Chinese.
-
Lipid Isobar and Isomer Imaging Using Nanospray Desorption Electrospray Ionization Combined with Triple Quadrupole Mass Spectrometry.Anal Chem. 2024 Feb 6. doi: 10.1021/acs.analchem.3c04705. Online ahead of print. Anal Chem. 2024. PMID: 38321595
-
Mass Spectrometry Imaging and Integration with Other Imaging Modalities for Greater Molecular Understanding of Biological Tissues.Mol Imaging Biol. 2018 Dec;20(6):888-901. doi: 10.1007/s11307-018-1267-y. Mol Imaging Biol. 2018. PMID: 30167993 Free PMC article. Review.
Cited by
-
Nanospray Desorption Electrospray Ionization Mass Spectrometry Imaging (nano-DESI MSI): A Tutorial Review.ACS Meas Sci Au. 2024 Aug 21;4(5):475-487. doi: 10.1021/acsmeasuresciau.4c00028. eCollection 2024 Oct 16. ACS Meas Sci Au. 2024. PMID: 39430971 Free PMC article. Review.
-
Spatial pharmacology using mass spectrometry imaging.Trends Pharmacol Sci. 2024 Jan;45(1):67-80. doi: 10.1016/j.tips.2023.11.003. Epub 2023 Dec 15. Trends Pharmacol Sci. 2024. PMID: 38103980 Review.
-
A monolithic microfluidic probe for ambient mass spectrometry imaging of biological tissues.Lab Chip. 2023 Oct 24;23(21):4664-4673. doi: 10.1039/d3lc00637a. Lab Chip. 2023. PMID: 37782224 Free PMC article.
-
Maximized Spatial Information and Minimized Acquisition Time of Top-Hat IR-MALDESI-MSI of Zebrafish Using Nested Regions of Interest (nROIs).J Am Soc Mass Spectrom. 2023 Sep 6;34(9):2043-2050. doi: 10.1021/jasms.3c00210. Epub 2023 Aug 1. J Am Soc Mass Spectrom. 2023. PMID: 37526449 Free PMC article.
-
Nanospray Desorption Electrospray Ionization (Nano-DESI) Mass Spectrometry Imaging with High Ion Mobility Resolution.J Am Soc Mass Spectrom. 2023 Aug 2;34(8):1798-1804. doi: 10.1021/jasms.3c00199. Epub 2023 Jul 18. J Am Soc Mass Spectrom. 2023. PMID: 37463098 Free PMC article.
References
-
- Swales JG, Hamm G, Clench MR, Goodwin RJA, Mass spectrometry imaging and its application in pharmaceutical research and development: A concise review, Int J Mass Spectrom. 437 (2019) 99–112. 10.1016/j.ijms.2018.02.007. - DOI
Grants and funding
LinkOut - more resources
Full Text Sources
Miscellaneous