This is a preprint.
Multimodal characterization of antigen-specific CD8 + T cells across SARS-CoV-2 vaccination and infection
- PMID: 36747786
- PMCID: PMC9900816
- DOI: 10.1101/2023.01.24.525203
Multimodal characterization of antigen-specific CD8 + T cells across SARS-CoV-2 vaccination and infection
Update in
-
Multimodal single-cell datasets characterize antigen-specific CD8+ T cells across SARS-CoV-2 vaccination and infection.Nat Immunol. 2023 Oct;24(10):1725-1734. doi: 10.1038/s41590-023-01608-9. Epub 2023 Sep 21. Nat Immunol. 2023. PMID: 37735591 Free PMC article.
Abstract
The human immune response to SARS-CoV-2 antigen after infection or vaccination is defined by the durable production of antibodies and T cells. Population-based monitoring typically focuses on antibody titer, but there is a need for improved characterization and quantification of T cell responses. Here, we utilize multimodal sequencing technologies to perform a longitudinal analysis of circulating human leukocytes collected before and after BNT162b2 immunization. Our data reveal distinct subpopulations of CD8 + T cells which reliably appear 28 days after prime vaccination (7 days post boost). Using a suite of cross-modality integration tools, we define their transcriptome, accessible chromatin landscape, and immunophenotype, and identify unique biomarkers within each modality. By leveraging DNA-oligo-tagged peptide-MHC multimers and T cell receptor sequencing, we demonstrate that this vaccine-induced population is SARS-CoV-2 antigen-specific and capable of rapid clonal expansion. Moreover, we also identify these CD8 + populations in scRNA-seq datasets from COVID-19 patients and find that their relative frequency and differentiation outcomes are predictive of subsequent clinical outcomes. Our work contributes to our understanding of T cell immunity, and highlights the potential for integrative and multimodal analysis to characterize rare cell populations.
Conflict of interest statement
Competing interests:
In the past three years, R.S. has worked as a consultant for Bristol-Myers Squibb, Regeneron, and Kallyope and served as an SAB member for ImmunAI, Resolve Biosciences, Nanostring, and the NYC Pandemic Response Lab. D.R.L. is cofounder of Vedanta Biosciences and ImmunAI, on the advisory boards of IMIDomics and Evommune, and on the board of directors of Pfizer. MJM reported potential competing interests: laboratory research and clinical trials contracts with Lilly, Pfizer (exclusive of the current work), and Sanofi for vaccines or MAB vs SARS-CoV-2; contract funding from USG/HHS/BARDA for research specimen characterization and repository; research grant funding from USG/HHS/NIH for SARS-CoV-2 vaccine and MAB clinical trials; personal fees from Meissa Vaccines, Inc. and Pfizer for Scientific Advisory Board service. RSH has received research support from CareDx for SARS-CoV-2 vaccine studies. RSH is a consultant for Bristol-Myers-Squibb. All other authors declare no competing interests.
Figures
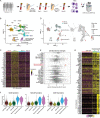
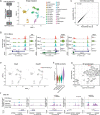
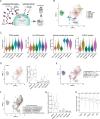
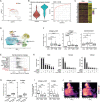
Similar articles
-
Multimodal single-cell datasets characterize antigen-specific CD8+ T cells across SARS-CoV-2 vaccination and infection.Nat Immunol. 2023 Oct;24(10):1725-1734. doi: 10.1038/s41590-023-01608-9. Epub 2023 Sep 21. Nat Immunol. 2023. PMID: 37735591 Free PMC article.
-
Tracking the immune response profiles elicited by the BNT162b2 vaccine in COVID-19 unexperienced and experienced individuals.Clin Immunol. 2024 Apr;261:110164. doi: 10.1016/j.clim.2024.110164. Epub 2024 Feb 28. Clin Immunol. 2024. PMID: 38417765
-
Transcriptomic profile of TNFhigh MAIT cells is linked to B cell response following SARS-CoV-2 vaccination.Front Immunol. 2023 Jul 26;14:1208662. doi: 10.3389/fimmu.2023.1208662. eCollection 2023. Front Immunol. 2023. PMID: 37564651 Free PMC article.
-
What are the roles of antibodies versus a durable, high quality T-cell response in protective immunity against SARS-CoV-2?Vaccine X. 2020 Dec 11;6:100076. doi: 10.1016/j.jvacx.2020.100076. Epub 2020 Aug 28. Vaccine X. 2020. PMID: 32875286 Free PMC article. Review.
-
Durable CD8 T Cell Memory against SARS-CoV-2 by Prime/Boost and Multi-Dose Vaccination: Considerations on Inter-Dose Time Intervals.Int J Mol Sci. 2022 Nov 19;23(22):14367. doi: 10.3390/ijms232214367. Int J Mol Sci. 2022. PMID: 36430845 Free PMC article. Review.
References
-
- Sahin U. et al. COVID-19 vaccine BNT162b1 elicits human antibody and TH1 T cell responses. Nature 586, 594–599 (2020). - PubMed
Publication types
Grants and funding
LinkOut - more resources
Full Text Sources
Research Materials
Miscellaneous