SARS-CoV-2 Omicron-B.1.1.529 leads to widespread escape from neutralizing antibody responses
- PMID: 35081335
- PMCID: PMC8723827
- DOI: 10.1016/j.cell.2021.12.046
SARS-CoV-2 Omicron-B.1.1.529 leads to widespread escape from neutralizing antibody responses
Abstract
On 24th November 2021, the sequence of a new SARS-CoV-2 viral isolate Omicron-B.1.1.529 was announced, containing far more mutations in Spike (S) than previously reported variants. Neutralization titers of Omicron by sera from vaccinees and convalescent subjects infected with early pandemic Alpha, Beta, Gamma, or Delta are substantially reduced, or the sera failed to neutralize. Titers against Omicron are boosted by third vaccine doses and are high in both vaccinated individuals and those infected by Delta. Mutations in Omicron knock out or substantially reduce neutralization by most of the large panel of potent monoclonal antibodies and antibodies under commercial development. Omicron S has structural changes from earlier viruses and uses mutations that confer tight binding to ACE2 to unleash evolution driven by immune escape. This leads to a large number of mutations in the ACE2 binding site and rebalances receptor affinity to that of earlier pandemic viruses.
Keywords: Omicron; RBD; SARS-CoV-2; Spike; immune evasion; receptor interaction; vaccines; variants.
Copyright © 2022 The Authors. Published by Elsevier Inc. All rights reserved.
Conflict of interest statement
Declaration of interests G.R.S. sits on the GSK Vaccines Scientific Advisory Board and is a founder member of RQ Biotechnology. J.Z. and G.S. declare the Israel patent application no. 23/09/2020—277,546 and United States patent application no. 16/12/2020—63/125,984, entitled methods and compositions for treating coronaviral infections. Oxford University holds intellectual property related to the Oxford-Astra Zeneca vaccine. A.J.P. is Chair of UK Dept. Health and Social Care’s (DHSC) Joint Committee on Vaccination & Immunisation (JCVI) but does not participate in the JCVI COVID-19 committee, and is a member of the WHO’s SAGE. The views expressed in this article do not necessarily represent the views of DHSC, JCVI, or WHO. The University of Oxford has entered into a partnership with AstraZeneca on coronavirus vaccine development. The University of Oxford has protected intellectual property disclosed in this publication. S.C.G. is co-founder of Vaccitech (collaborators in the early development of this vaccine candidate) and is named as an inventor on a patent covering use of ChAdOx1-vectored vaccines and a patent application covering this SARS-CoV-2 vaccine (PCT/GB2012/000467). T.L. is named as an inventor on a patent application covering this SARS-CoV-2 vaccine and was a consultant to Vaccitech for an unrelated project during the conduct of the study. S.J.D. is a Scientific Advisor to the Scottish Parliament on COVID-19.
Figures
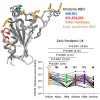
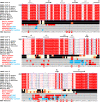
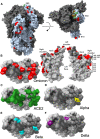
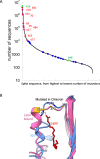
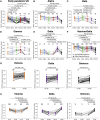
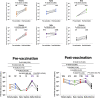
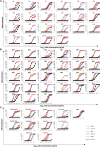
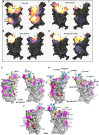
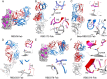
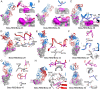

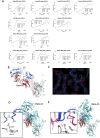
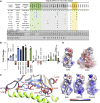
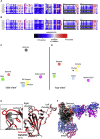
Update of
-
Omicron-B.1.1.529 leads to widespread escape from neutralizing antibody responses.bioRxiv [Preprint]. 2021 Dec 22:2021.12.03.471045. doi: 10.1101/2021.12.03.471045. bioRxiv. 2021. Update in: Cell. 2022 Feb 3;185(3):467-484.e15. doi: 10.1016/j.cell.2021.12.046 PMID: 34981049 Free PMC article. Updated. Preprint.
Comment in
-
Omicron, the great escape artist.Nat Rev Immunol. 2022 Feb;22(2):75. doi: 10.1038/s41577-022-00676-6. Nat Rev Immunol. 2022. PMID: 35017722 Free PMC article.
-
Omicron's message on vaccines: Boosting begets breadth.Cell. 2022 Feb 3;185(3):411-413. doi: 10.1016/j.cell.2022.01.006. Epub 2022 Jan 14. Cell. 2022. PMID: 35065712 Free PMC article.
-
Beyond gut instincts: Microbe survival depends on sugars and butyrate.Cell. 2022 Feb 3;185(3):414-416. doi: 10.1016/j.cell.2021.12.037. Cell. 2022. PMID: 35120661
Similar articles
-
Omicron-B.1.1.529 leads to widespread escape from neutralizing antibody responses.bioRxiv [Preprint]. 2021 Dec 22:2021.12.03.471045. doi: 10.1101/2021.12.03.471045. bioRxiv. 2021. Update in: Cell. 2022 Feb 3;185(3):467-484.e15. doi: 10.1016/j.cell.2021.12.046 PMID: 34981049 Free PMC article. Updated. Preprint.
-
Safety and immunogenicity of a modified mRNA-lipid nanoparticle vaccine candidate against COVID-19: Results from a phase 1, dose-escalation study.Hum Vaccin Immunother. 2024 Dec 31;20(1):2408863. doi: 10.1080/21645515.2024.2408863. Epub 2024 Oct 18. Hum Vaccin Immunother. 2024. PMID: 39422261 Free PMC article. Clinical Trial.
-
Deep mutational scans for ACE2 binding, RBD expression, and antibody escape in the SARS-CoV-2 Omicron BA.1 and BA.2 receptor-binding domains.PLoS Pathog. 2022 Nov 18;18(11):e1010951. doi: 10.1371/journal.ppat.1010951. eCollection 2022 Nov. PLoS Pathog. 2022. PMID: 36399443 Free PMC article.
-
Depressing time: Waiting, melancholia, and the psychoanalytic practice of care.In: Kirtsoglou E, Simpson B, editors. The Time of Anthropology: Studies of Contemporary Chronopolitics. Abingdon: Routledge; 2020. Chapter 5. In: Kirtsoglou E, Simpson B, editors. The Time of Anthropology: Studies of Contemporary Chronopolitics. Abingdon: Routledge; 2020. Chapter 5. PMID: 36137063 Free Books & Documents. Review.
-
Trends in Surgical and Nonsurgical Aesthetic Procedures: A 14-Year Analysis of the International Society of Aesthetic Plastic Surgery-ISAPS.Aesthetic Plast Surg. 2024 Oct;48(20):4217-4227. doi: 10.1007/s00266-024-04260-2. Epub 2024 Aug 5. Aesthetic Plast Surg. 2024. PMID: 39103642 Review.
Cited by
-
Effectiveness of a broad-spectrum bivalent mRNA vaccine against SARS-CoV-2 variants in preclinical studies.Emerg Microbes Infect. 2024 Dec;13(1):2321994. doi: 10.1080/22221751.2024.2321994. Epub 2024 Feb 29. Emerg Microbes Infect. 2024. PMID: 38377136 Free PMC article.
-
The efficacy of paxlovid in elderly patients infected with SARS-CoV-2 omicron variants: Results of a non-randomized clinical trial.Front Med (Lausanne). 2022 Sep 6;9:980002. doi: 10.3389/fmed.2022.980002. eCollection 2022. Front Med (Lausanne). 2022. PMID: 36148451 Free PMC article.
-
SARS-CoV-2 reinfections during the Delta and Omicron waves.JCI Insight. 2022 Oct 24;7(20):e162007. doi: 10.1172/jci.insight.162007. JCI Insight. 2022. PMID: 36048527 Free PMC article.
-
Antigen presentation dynamics shape the response to emergent variants like SARS-CoV-2 Omicron strain after multiple vaccinations with wild type strain.bioRxiv [Preprint]. 2022 Aug 25:2022.08.24.505127. doi: 10.1101/2022.08.24.505127. bioRxiv. 2022. Update in: Cell Rep. 2023 Apr 25;42(4):112256. doi: 10.1016/j.celrep.2023.112256 PMID: 36052368 Free PMC article. Updated. Preprint.
-
Efficacy of convalescent plasma in hospitalized COVID-19 patients: findings from a controlled trial.Braz J Med Biol Res. 2024 Oct 7;57:e13627. doi: 10.1590/1414-431X2024e13627. eCollection 2024. Braz J Med Biol Res. 2024. PMID: 39383382 Free PMC article. Clinical Trial.
References
-
- Acharya R., Fry E., Stuart D., Fox G., Rowlands D., Brown F. The three-dimensional structure of foot-and-mouth disease virus at 2.9 A resolution. Nature. 1989;337:709–716. - PubMed
-
- Aricescu A.R., Lu W., Jones E.Y. A time- and cost-efficient system for high-level protein production in mammalian cells. Acta Crystallogr. D Biol. Crystallogr. 2006;62:1243–1250. - PubMed
-
- Caly L., Druce J., Roberts J., Bond K., Tran T., Kostecki R., Yoga Y., Naughton W., Taiaroa G., Seemann T., et al. Isolation and rapid sharing of the 2019 novel coronavirus (SARS-CoV-2) from the first patient diagnosed with COVID-19 in Australia. Med. J. Aust. 2020;212:459–462. doi: 10.5694/mja2.50569. - DOI - PMC - PubMed
Grants and funding
- 203224/Z/16/Z/WT_/Wellcome Trust/United Kingdom
- MC_PC_19059/MRC_/Medical Research Council/United Kingdom
- COV/GLA/20/05/CSO_/Chief Scientist Office/United Kingdom
- MR/P011705/2/MRC_/Medical Research Council/United Kingdom
- MR/V001329/1/MRC_/Medical Research Council/United Kingdom
- MR/S032304/1/MRC_/Medical Research Council/United Kingdom
- WT_/Wellcome Trust/United Kingdom
- MC_UP_A090_1006/MRC_/Medical Research Council/United Kingdom
- MR/S020039/1/MRC_/Medical Research Council/United Kingdom
- MR/N00065X/1/MRC_/Medical Research Council/United Kingdom
- MC_PC_19025/MRC_/Medical Research Council/United Kingdom
- MR/P011705/1/MRC_/Medical Research Council/United Kingdom
LinkOut - more resources
Full Text Sources
Other Literature Sources
Miscellaneous