Differential splicing of the lectin domain of an O-glycosyltransferase modulates both peptide and glycopeptide preferences
- PMID: 32669364
- PMCID: PMC7458804
- DOI: 10.1074/jbc.RA120.014700
Differential splicing of the lectin domain of an O-glycosyltransferase modulates both peptide and glycopeptide preferences
Abstract
Mucin-type O-glycosylation is an essential post-translational modification required for protein secretion, extracellular matrix formation, and organ growth. O-Glycosylation is initiated by a large family of enzymes (GALNTs in mammals and PGANTs in Drosophila) that catalyze the addition of GalNAc onto the hydroxyl groups of serines or threonines in protein substrates. These enzymes contain two functional domains: a catalytic domain and a C-terminal ricin-like lectin domain comprised of three potential GalNAc recognition repeats termed α, β, and γ. The catalytic domain is responsible for binding donor and acceptor substrates and catalyzing transfer of GalNAc, whereas the lectin domain recognizes more distant extant GalNAc on previously glycosylated substrates. We previously demonstrated a novel role for the α repeat of lectin domain in influencing charged peptide preferences. Here, we further interrogate how the differentially spliced α repeat of the PGANT9A and PGANT9B O-glycosyltransferases confers distinct preferences for a variety of endogenous substrates. Through biochemical analyses and in silico modeling using preferred substrates, we find that a combination of charged residues within the α repeat and charged residues in the flexible gating loop of the catalytic domain distinctively influence the peptide substrate preferences of each splice variant. Moreover, PGANT9A and PGANT9B also display unique glycopeptide preferences. These data illustrate how changes within the noncatalytic lectin domain can alter the recognition of both peptide and glycopeptide substrates. Overall, our results elucidate a novel mechanism for modulating substrate preferences of O-glycosyltransferases via alternative splicing within specific subregions of functional domains.
Keywords: Drosophila; Galnt; O-glycosylation; PGANT; alternative splicing; lectin; lectin domain; mucin; salivary gland; secretion; splicing.
© 2020 May et al.
Conflict of interest statement
Conflict of interest—The authors declare that they have no conflicts of interest with the contents of this article.
Figures
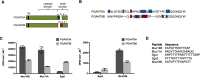
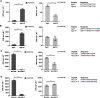
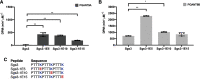
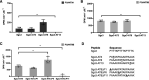
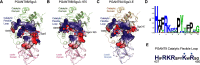
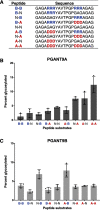
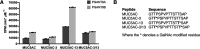
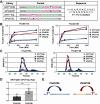
Similar articles
-
The lectin domain of the polypeptide GalNAc transferase family of glycosyltransferases (ppGalNAc Ts) acts as a switch directing glycopeptide substrate glycosylation in an N- or C-terminal direction, further controlling mucin type O-glycosylation.J Biol Chem. 2013 Jul 5;288(27):19900-14. doi: 10.1074/jbc.M113.477877. Epub 2013 May 20. J Biol Chem. 2013. PMID: 23689369 Free PMC article.
-
Mucin-type O-glycosylation is controlled by short- and long-range glycopeptide substrate recognition that varies among members of the polypeptide GalNAc transferase family.Glycobiology. 2016 Apr;26(4):360-76. doi: 10.1093/glycob/cwv108. Epub 2015 Nov 26. Glycobiology. 2016. PMID: 26610890 Free PMC article.
-
The lectin domains of polypeptide GalNAc-transferases exhibit carbohydrate-binding specificity for GalNAc: lectin binding to GalNAc-glycopeptide substrates is required for high density GalNAc-O-glycosylation.Glycobiology. 2007 Apr;17(4):374-87. doi: 10.1093/glycob/cwl082. Epub 2007 Jan 10. Glycobiology. 2007. PMID: 17215257
-
Polypeptide GalNAc-Ts: from redundancy to specificity.Curr Opin Struct Biol. 2019 Jun;56:87-96. doi: 10.1016/j.sbi.2018.12.007. Epub 2019 Jan 28. Curr Opin Struct Biol. 2019. PMID: 30703750 Free PMC article. Review.
-
Molecular Recognition of GalNAc in Mucin-Type O-Glycosylation.Acc Chem Res. 2023 Mar 7;56(5):548-560. doi: 10.1021/acs.accounts.2c00723. Epub 2023 Feb 23. Acc Chem Res. 2023. PMID: 36815693 Free PMC article. Review.
Cited by
-
Aberrant glycosylation in schizophrenia: insights into pathophysiological mechanisms and therapeutic potentials.Front Pharmacol. 2024 Sep 2;15:1457811. doi: 10.3389/fphar.2024.1457811. eCollection 2024. Front Pharmacol. 2024. PMID: 39286629 Free PMC article. Review.
-
Polypeptide N-acetylgalactosaminyltransferase (GalNAc-T) isozyme surface charge governs charge substrate preferences to modulate mucin type O-glycosylation.Glycobiology. 2023 Oct 30;33(10):817-836. doi: 10.1093/glycob/cwad066. Glycobiology. 2023. PMID: 37555669 Free PMC article.
-
Transcriptional changes in specific subsets of Drosophila neurons following inhibition of the serotonin transporter.Transl Psychiatry. 2023 Jun 24;13(1):226. doi: 10.1038/s41398-023-02521-3. Transl Psychiatry. 2023. PMID: 37355701 Free PMC article.
-
Transcriptional changes in specific subsets of Drosophila neurons following inhibition of the serotonin transporter.Res Sq [Preprint]. 2023 Mar 17:rs.3.rs-2626506. doi: 10.21203/rs.3.rs-2626506/v1. Res Sq. 2023. Update in: Transl Psychiatry. 2023 Jun 24;13(1):226. doi: 10.1038/s41398-023-02521-3. PMID: 36993644 Free PMC article. Updated. Preprint.
-
Polypeptide N-acetylgalactosamine transferase 3: a post-translational writer on human health.J Mol Med (Berl). 2022 Oct;100(10):1387-1403. doi: 10.1007/s00109-022-02249-5. Epub 2022 Sep 2. J Mol Med (Berl). 2022. PMID: 36056254 Review.
References
-
- Topaz O., Shurman D. L., Bergman R., Indelman M., Ratajczak P., Mizrachi M., Khamaysi Z., Behar D., Petronius D., Friedman V., Zelikovic I., Raimer S., Metzker A., Richard G., and Sprecher E. (2004) Mutations in GALNT3, encoding a protein involved in O-linked glycosylation, cause familial tumoral calcinosis. Nat. Genet. 36, 579–581 10.1038/ng1358 - DOI - PubMed
-
- Ichikawa S., Sorenson A. H., Austin A. M., Mackenzie D. S., Fritz T. A., Moh A., Hui S. L., and Econs M. J. (2009) Ablation of the Galnt3 gene leads to low-circulating intact fibroblast growth factor 23 (Fgf23) concentrations and hyperphosphatemia despite increased Fgf23 expression. Endocrinology 150, 2543–2550 10.1210/en.2008-0877 - DOI - PMC - PubMed
-
- Guda K., Moinova H., He J., Jamison O., Ravi L., Natale L., Lutterbaugh J., Lawrence E., Lewis S., Willson J. K., Lowe J. B., Wiesner G. L., Parmigiani G., Barnholtz-Sloan J., Dawson D. W., et al. (2009) Inactivating germ-line and somatic mutations in polypeptide N-acetylgalactosaminyltransferase 12 in human colon cancers. Proc. Natl. Acad. Sci. U.S.A. 106, 12921–12925 10.1073/pnas.0901454106 - DOI - PMC - PubMed
Publication types
MeSH terms
Substances
Associated data
- Actions
- Actions
- Actions
- Actions
Grants and funding
LinkOut - more resources
Full Text Sources
Molecular Biology Databases