Comprehensive Dual- and Triple-Feature Intersectional Single-Vector Delivery of Diverse Functional Payloads to Cells of Behaving Mammals
- PMID: 32574559
- PMCID: PMC7687746
- DOI: 10.1016/j.neuron.2020.06.003
Comprehensive Dual- and Triple-Feature Intersectional Single-Vector Delivery of Diverse Functional Payloads to Cells of Behaving Mammals
Abstract
The resolution and dimensionality with which biologists can characterize cell types have expanded dramatically in recent years, and intersectional consideration of such features (e.g., multiple gene expression and anatomical parameters) is increasingly understood to be essential. At the same time, genetically targeted technology for writing in and reading out activity patterns for cells in living organisms has enabled causal investigation in physiology and behavior; however, cell-type-specific delivery of these tools (including microbial opsins for optogenetics and genetically encoded Ca2+ indicators) has thus far fallen short of versatile targeting to cells jointly defined by many individually selected features. Here, we develop a comprehensive intersectional targeting toolbox including 39 novel vectors for joint-feature-targeted delivery of 13 molecular payloads (including opsins, indicators, and fluorophores), systematic approaches for development and optimization of new intersectional tools, hardware for in vivo monitoring of expression dynamics, and the first versatile single-virus tools (Triplesect) that enable targeting of triply defined cell types.
Keywords: Cre; Flp; VCre; calcium imaging; channelrhodopsin; fluorophores; intersectional; optogenetics; recombinase; targeting.
Copyright © 2020 Elsevier Inc. All rights reserved.
Conflict of interest statement
Declaration of Interests The authors declare no competing interests.
Figures
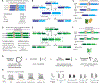
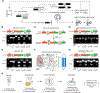
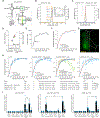
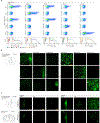
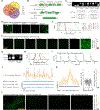
Similar articles
-
tTARGIT AAVs mediate the sensitive and flexible manipulation of intersectional neuronal populations in mice.Elife. 2021 Mar 11;10:e66835. doi: 10.7554/eLife.66835. Elife. 2021. PMID: 33704065 Free PMC article.
-
A CRISPR toolbox for generating intersectional genetic mouse models for functional, molecular, and anatomical circuit mapping.BMC Biol. 2022 Jan 28;20(1):28. doi: 10.1186/s12915-022-01227-0. BMC Biol. 2022. PMID: 35086530 Free PMC article.
-
An intein-split transactivator for intersectional neural imaging and optogenetic manipulation.Nat Commun. 2022 Jun 23;13(1):3605. doi: 10.1038/s41467-022-31255-x. Nat Commun. 2022. PMID: 35739125 Free PMC article.
-
Challenges for Therapeutic Applications of Opsin-Based Optogenetic Tools in Humans.Front Neural Circuits. 2020 Jul 15;14:41. doi: 10.3389/fncir.2020.00041. eCollection 2020. Front Neural Circuits. 2020. PMID: 32760252 Free PMC article. Review.
-
Optogenetics: Illuminating the Future of Hearing Restoration and Understanding Auditory Perception.Curr Gene Ther. 2024;24(3):208-216. doi: 10.2174/0115665232269742231213110937. Curr Gene Ther. 2024. PMID: 38676313 Review.
Cited by
-
Long-term optical imaging of the spinal cord in awake behaving mice.Nat Methods. 2024 Dec;21(12):2363-2375. doi: 10.1038/s41592-024-02476-3. Epub 2024 Nov 12. Nat Methods. 2024. PMID: 39533007
-
Monosynaptic Inputs to Ventral Tegmental Area Glutamate and GABA Co-transmitting Neurons.J Neurosci. 2024 Nov 13;44(46):e2184232024. doi: 10.1523/JNEUROSCI.2184-23.2024. J Neurosci. 2024. PMID: 39327007
-
Neuromodulator and neuropeptide sensors and probes for precise circuit interrogation in vivo.Science. 2024 Sep 27;385(6716):eadn6671. doi: 10.1126/science.adn6671. Epub 2024 Sep 27. Science. 2024. PMID: 39325905 Free PMC article. Review.
-
Adrenergic C1 neurons enhance anxiety via projections to PAG.bioRxiv [Preprint]. 2024 Sep 12:2024.09.11.612440. doi: 10.1101/2024.09.11.612440. bioRxiv. 2024. PMID: 39314285 Free PMC article. Preprint.
-
Identifying dysfunctional cell types and circuits in animal models for psychiatric disorders with calcium imaging.Neuropsychopharmacology. 2024 Nov;50(1):274-284. doi: 10.1038/s41386-024-01942-y. Epub 2024 Aug 9. Neuropsychopharmacology. 2024. PMID: 39122815 Review.
References
-
- Andrews BJ, Proteau GA, Beatty LG, and Sadowski PD (1985). The FLP recombinase of the 2μ circle DNA of yeast: Interaction with its target sequences. Cell 40, 795–803. - PubMed
-
- Asp M, Giacomello S, Larsson L, Wu C, Fürth D, Qian X, Wärdell E, Custodio J, Reimegård J, Salmén F, et al. (2019). A Spatiotemporal Organ-Wide Gene Expression and Cell Atlas of the Developing Human Heart. Cell 179, 1647–1660.e19. - PubMed
Publication types
MeSH terms
Grants and funding
LinkOut - more resources
Full Text Sources
Other Literature Sources
Molecular Biology Databases
Research Materials
Miscellaneous