The Sensory Coding of Warm Perception
- PMID: 32208171
- PMCID: PMC7272120
- DOI: 10.1016/j.neuron.2020.02.035
The Sensory Coding of Warm Perception
Abstract
Humans detect skin temperature changes that are perceived as warm or cool. Like humans, mice report forepaw skin warming with perceptual thresholds of less than 1°C and do not confuse warm with cool. We identify two populations of polymodal C-fibers that signal warm. Warm excites one population, whereas it suppresses the ongoing cool-driven firing of the other. In the absence of the thermosensitive TRPM2 or TRPV1 ion channels, warm perception was blunted, but not abolished. In addition, trpv1:trpa1:trpm3-/- triple-mutant mice that cannot sense noxious heat detected skin warming, albeit with reduced sensitivity. In contrast, loss or local pharmacological silencing of the cool-driven TRPM8 channel abolished the ability to detect warm. Our data are not reconcilable with a labeled line model for warm perception, with receptors firing only in response to warm stimuli, but instead support a conserved dual sensory model to unambiguously detect skin warming in vertebrates.
Keywords: C-fiber; Trp channels; nociception; perception; polymodal; sensory coding; thermal transduction; warm.
Copyright © 2020 The Author(s). Published by Elsevier Inc. All rights reserved.
Conflict of interest statement
Declaration of Interests The authors declare no competing interests.
Figures
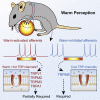
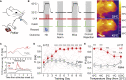
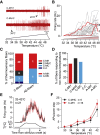
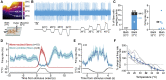
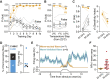
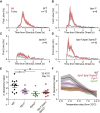
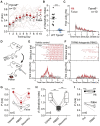
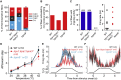
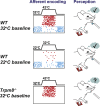
Comment in
-
Feeling the warmth.Nat Rev Neurosci. 2020 Jun;21(6):299. doi: 10.1038/s41583-020-0302-6. Nat Rev Neurosci. 2020. PMID: 32246102 No abstract available.
-
Detecting Warm Temperatures Is a Cool Kind of Thing.Neuron. 2020 Jun 3;106(5):712-714. doi: 10.1016/j.neuron.2020.05.009. Neuron. 2020. PMID: 32497507
Similar articles
-
A TRP channel trio mediates acute noxious heat sensing.Nature. 2018 Mar 29;555(7698):662-666. doi: 10.1038/nature26137. Epub 2018 Mar 14. Nature. 2018. PMID: 29539642
-
Thermosensation and TRP Channels.Adv Exp Med Biol. 2024;1461:3-13. doi: 10.1007/978-981-97-4584-5_1. Adv Exp Med Biol. 2024. PMID: 39289270 Review.
-
Cav3.2-expressing low-threshold C fibres in human hairy skin contribute to cold allodynia--a non-TRPV1- and non-TRPM8-dependent phenomenon.Pain. 2015 Aug;156(8):1566-1575. doi: 10.1097/j.pain.0000000000000202. Pain. 2015. PMID: 25932689
-
Role of thermosensitive transient receptor potential (TRP) channels in thermal preference of male and female mice.J Therm Biol. 2024 May;122:103868. doi: 10.1016/j.jtherbio.2024.103868. Epub 2024 Jun 4. J Therm Biol. 2024. PMID: 38852485 Free PMC article.
-
[Molecular mechanisms of thermosensation].Nihon Yakurigaku Zasshi. 2004 Oct;124(4):219-27. doi: 10.1254/fpj.124.219. Nihon Yakurigaku Zasshi. 2004. PMID: 15467255 Review. Japanese.
Cited by
-
Regulation of transient receptor potential channels by traditional Chinese medicines and their active ingredients.Front Pharmacol. 2022 Oct 13;13:1039412. doi: 10.3389/fphar.2022.1039412. eCollection 2022. Front Pharmacol. 2022. PMID: 36313301 Free PMC article. Review.
-
Glial functions in the blood-brain communication at the circumventricular organs.Front Neurosci. 2022 Oct 6;16:991779. doi: 10.3389/fnins.2022.991779. eCollection 2022. Front Neurosci. 2022. PMID: 36278020 Free PMC article. Review.
-
Negative Modulation of TRPM8 Channel Function by Protein Kinase C in Trigeminal Cold Thermoreceptor Neurons.Int J Mol Sci. 2020 Jun 22;21(12):4420. doi: 10.3390/ijms21124420. Int J Mol Sci. 2020. PMID: 32580281 Free PMC article.
-
Optical Assessment of Nociceptive TRP Channel Function at the Peripheral Nerve Terminal.Int J Mol Sci. 2021 Jan 6;22(2):481. doi: 10.3390/ijms22020481. Int J Mol Sci. 2021. PMID: 33418928 Free PMC article. Review.
-
An operant temperature sensory assay provides a means to assess thermal discrimination.Mol Pain. 2021 Jan-Dec;17:17448069211013633. doi: 10.1177/17448069211013633. Mol Pain. 2021. PMID: 33906493 Free PMC article.
References
-
- Bautista D.M., Siemens J., Glazer J.M., Tsuruda P.R., Basbaum A.I., Stucky C.L., Jordt S.-E.E., Julius D. The menthol receptor TRPM8 is the principal detector of environmental cold. Nature. 2007;448:204–208. - PubMed
-
- Belmonte C., Brock J.A., Viana F. Converting cold into pain. Exp. Brain Res. 2009;196:13–30. - PubMed
-
- Blix M. Experimentela bidrag till losning af fragan om hudnervernas specifika energi. Uppsala Lakfor. Forh. 1882;18:87–102.
-
- Bubb M.R., Senderowicz A.M., Sausville E.A., Duncan K.L., Korn E.D. Jasplakinolide, a cytotoxic natural product, induces actin polymerization and competitively inhibits the binding of phalloidin to F-actin. J. Biol. Chem. 1994;269:14869–14871. - PubMed
Publication types
MeSH terms
Substances
LinkOut - more resources
Full Text Sources
Other Literature Sources
Molecular Biology Databases
Research Materials