A genetically encoded fluorescent acetylcholine indicator for in vitro and in vivo studies
- PMID: 29985477
- PMCID: PMC6093211
- DOI: 10.1038/nbt.4184
A genetically encoded fluorescent acetylcholine indicator for in vitro and in vivo studies
Abstract
The neurotransmitter acetylcholine (ACh) regulates a diverse array of physiological processes throughout the body. Despite its importance, cholinergic transmission in the majority of tissues and organs remains poorly understood owing primarily to the limitations of available ACh-monitoring techniques. We developed a family of ACh sensors (GACh) based on G-protein-coupled receptors that has the sensitivity, specificity, signal-to-noise ratio, kinetics and photostability suitable for monitoring ACh signals in vitro and in vivo. GACh sensors were validated with transfection, viral and/or transgenic expression in a dozen types of neuronal and non-neuronal cells prepared from multiple animal species. In all preparations, GACh sensors selectively responded to exogenous and/or endogenous ACh with robust fluorescence signals that were captured by epifluorescence, confocal, and/or two-photon microscopy. Moreover, analysis of endogenous ACh release revealed firing-pattern-dependent release and restricted volume transmission, resolving two long-standing questions about central cholinergic transmission. Thus, GACh sensors provide a user-friendly, broadly applicable tool for monitoring cholinergic transmission underlying diverse biological processes.
Figures
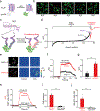
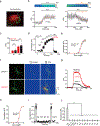
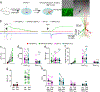
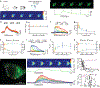
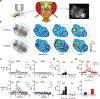
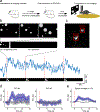
Similar articles
-
An optimized acetylcholine sensor for monitoring in vivo cholinergic activity.Nat Methods. 2020 Nov;17(11):1139-1146. doi: 10.1038/s41592-020-0953-2. Epub 2020 Sep 28. Nat Methods. 2020. PMID: 32989318 Free PMC article.
-
Comparison of fluorescence biosensors and whole-cell patch clamp recording in detecting ACh, NE, and 5-HT.Front Cell Neurosci. 2023 Jun 2;17:1166480. doi: 10.3389/fncel.2023.1166480. eCollection 2023. Front Cell Neurosci. 2023. PMID: 37333890 Free PMC article.
-
How can I measure brain acetylcholine levels in vivo? Advantages and caveats of commonly used approaches.J Neurochem. 2023 Oct;167(1):3-15. doi: 10.1111/jnc.15943. Epub 2023 Aug 24. J Neurochem. 2023. PMID: 37621094 Free PMC article. Review.
-
Evoked acetylcholine release by immortalized brain endothelial cells genetically modified to express choline acetyltransferase and/or the vesicular acetylcholine transporter.J Neurochem. 1999 Oct;73(4):1483-91. doi: 10.1046/j.1471-4159.1999.0731483.x. J Neurochem. 1999. PMID: 10501193
-
Cotransmission of acetylcholine and GABA.Neuropharmacology. 2016 Jan;100:40-6. doi: 10.1016/j.neuropharm.2015.07.031. Epub 2015 Jul 26. Neuropharmacology. 2016. PMID: 26220313 Free PMC article. Review.
Cited by
-
Non-Invasive On-Off Fluorescent Biosensor for Endothelial Cell Detection.Biosensors (Basel). 2024 Oct 9;14(10):489. doi: 10.3390/bios14100489. Biosensors (Basel). 2024. PMID: 39451702 Free PMC article.
-
Neuromodulator and neuropeptide sensors and probes for precise circuit interrogation in vivo.Science. 2024 Sep 27;385(6716):eadn6671. doi: 10.1126/science.adn6671. Epub 2024 Sep 27. Science. 2024. PMID: 39325905 Free PMC article. Review.
-
Fiber photometry in neuroscience research: principles, applications, and future directions.Pharmacol Rep. 2024 Dec;76(6):1242-1255. doi: 10.1007/s43440-024-00646-w. Epub 2024 Sep 5. Pharmacol Rep. 2024. PMID: 39235662 Free PMC article. Review.
-
Ngfr+ cholinergic projection from SI/nBM to mPFC selectively regulates temporal order recognition memory.Nat Commun. 2024 Aug 26;15(1):7342. doi: 10.1038/s41467-024-51707-w. Nat Commun. 2024. PMID: 39187496 Free PMC article.
-
Cortical Acetylcholine Response to Deep Brain Stimulation of the Basal Forebrain.bioRxiv [Preprint]. 2024 Jul 31:2024.07.30.605828. doi: 10.1101/2024.07.30.605828. bioRxiv. 2024. PMID: 39131297 Free PMC article. Preprint.
References
-
- Dani JA & Bertrand D Nicotinic acetylcholine receptors and nicotinic cholinergic mechanisms of the central nervous system. Annual review of pharmacology and toxicology 47, 699–729 (2007). - PubMed
Publication types
MeSH terms
Substances
Grants and funding
LinkOut - more resources
Full Text Sources
Other Literature Sources
Molecular Biology Databases
Research Materials