The Molecular Basis for Ubiquitin and Ubiquitin-like Specificities in Bacterial Effector Proteases
- PMID: 27425412
- PMCID: PMC4961225
- DOI: 10.1016/j.molcel.2016.06.015
The Molecular Basis for Ubiquitin and Ubiquitin-like Specificities in Bacterial Effector Proteases
Abstract
Pathogenic bacteria rely on secreted effector proteins to manipulate host signaling pathways, often in creative ways. CE clan proteases, specific hydrolases for ubiquitin-like modifications (SUMO and NEDD8) in eukaryotes, reportedly serve as bacterial effector proteins with deSUMOylase, deubiquitinase, or, even, acetyltransferase activities. Here, we characterize bacterial CE protease activities, revealing K63-linkage-specific deubiquitinases in human pathogens, such as Salmonella, Escherichia, and Shigella, as well as ubiquitin/ubiquitin-like cross-reactive enzymes in Chlamydia, Rickettsia, and Xanthomonas. Five crystal structures, including ubiquitin/ubiquitin-like complexes, explain substrate specificities and redefine relationships across the CE clan. Importantly, this work identifies novel family members and provides key discoveries among previously reported effectors, such as the unexpected deubiquitinase activity in Xanthomonas XopD, contributed by an unstructured ubiquitin binding region. Furthermore, accessory domains regulate properties such as subcellular localization, as exemplified by a ubiquitin-binding domain in Salmonella Typhimurium SseL. Our work both highlights and explains the functional adaptations observed among diverse CE clan proteins.
Copyright © 2016 The Authors. Published by Elsevier Inc. All rights reserved.
Figures
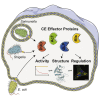
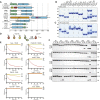
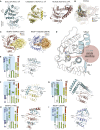
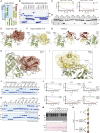
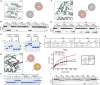
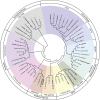
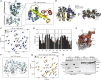
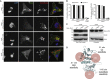
Similar articles
-
Identification of Salmonella Typhimurium Deubiquitinase SseL Substrates by Immunoaffinity Enrichment and Quantitative Proteomic Analysis.J Proteome Res. 2015 Sep 4;14(9):4029-38. doi: 10.1021/acs.jproteome.5b00574. Epub 2015 Aug 6. J Proteome Res. 2015. PMID: 26147956 Free PMC article.
-
ElaD, a Deubiquitinating protease expressed by E. coli.PLoS One. 2007 Apr 18;2(4):e381. doi: 10.1371/journal.pone.0000381. PLoS One. 2007. PMID: 17440617 Free PMC article.
-
Identification and characterization of diverse OTU deubiquitinases in bacteria.EMBO J. 2020 Aug 3;39(15):e105127. doi: 10.15252/embj.2020105127. Epub 2020 Jun 22. EMBO J. 2020. PMID: 32567101 Free PMC article.
-
Bacterial usurpation of the OTU deubiquitinase fold.FEBS J. 2024 Aug;291(15):3303-3316. doi: 10.1111/febs.16725. Epub 2023 Jan 24. FEBS J. 2024. PMID: 36636866 Free PMC article. Review.
-
Exploitation of the host cell ubiquitin machinery by microbial effector proteins.J Cell Sci. 2017 Jun 15;130(12):1985-1996. doi: 10.1242/jcs.188482. Epub 2017 May 5. J Cell Sci. 2017. PMID: 28476939 Free PMC article. Review.
Cited by
-
Divergence of Legionella Effectors Reversing Conventional and Unconventional Ubiquitination.Front Cell Infect Microbiol. 2020 Aug 21;10:448. doi: 10.3389/fcimb.2020.00448. eCollection 2020. Front Cell Infect Microbiol. 2020. PMID: 32974222 Free PMC article. Review.
-
Structural Basis of Substrate Recognition and Covalent Inhibition of Cdu1 from Chlamydia trachomatis.ChemMedChem. 2018 Oct 8;13(19):2014-2023. doi: 10.1002/cmdc.201800364. Epub 2018 Sep 6. ChemMedChem. 2018. PMID: 30028574 Free PMC article.
-
Interaction of the Ankyrin H Core Effector of Legionella with the Host LARP7 Component of the 7SK snRNP Complex.mBio. 2019 Aug 27;10(4):e01942-19. doi: 10.1128/mBio.01942-19. mBio. 2019. PMID: 31455655 Free PMC article.
-
Evasion of autophagy mediated by Rickettsia surface protein OmpB is critical for virulence.Nat Microbiol. 2019 Dec;4(12):2538-2551. doi: 10.1038/s41564-019-0583-6. Epub 2019 Oct 14. Nat Microbiol. 2019. PMID: 31611642 Free PMC article.
-
Structural and functional study of Legionella pneumophila effector RavA.Protein Sci. 2021 May;30(5):940-955. doi: 10.1002/pro.4057. Epub 2021 Mar 9. Protein Sci. 2021. PMID: 33660322 Free PMC article.
References
-
- Ashida H., Kim M., Sasakawa C. Exploitation of the host ubiquitin system by human bacterial pathogens. Nat. Rev. Microbiol. 2014;12:399–413. - PubMed
-
- Auweter S.D., Yu H.B., Arena E.T., Guttman J.A., Finlay B.B. Oxysterol-binding protein (OSBP) enhances replication of intracellular Salmonella and binds the Salmonella SPI-2 effector SseL via its N-terminus. Microbes Infect. 2012;14:148–154. - PubMed
-
- Basters A., Geurink P.P., El Oualid F., Ketscher L., Casutt M.S., Krause E., Ovaa H., Knobeloch K.P., Fritz G. Molecular characterization of ubiquitin-specific protease 18 reveals substrate specificity for interferon-stimulated gene 15. FEBS J. 2014;281:1918–1928. - PubMed
MeSH terms
Substances
Grants and funding
LinkOut - more resources
Full Text Sources
Other Literature Sources
Miscellaneous