CTCF-Mediated Human 3D Genome Architecture Reveals Chromatin Topology for Transcription
- PMID: 26686651
- PMCID: PMC4734140
- DOI: 10.1016/j.cell.2015.11.024
CTCF-Mediated Human 3D Genome Architecture Reveals Chromatin Topology for Transcription
Abstract
Spatial genome organization and its effect on transcription remains a fundamental question. We applied an advanced chromatin interaction analysis by paired-end tag sequencing (ChIA-PET) strategy to comprehensively map higher-order chromosome folding and specific chromatin interactions mediated by CCCTC-binding factor (CTCF) and RNA polymerase II (RNAPII) with haplotype specificity and nucleotide resolution in different human cell lineages. We find that CTCF/cohesin-mediated interaction anchors serve as structural foci for spatial organization of constitutive genes concordant with CTCF-motif orientation, whereas RNAPII interacts within these structures by selectively drawing cell-type-specific genes toward CTCF foci for coordinated transcription. Furthermore, we show that haplotype variants and allelic interactions have differential effects on chromosome configuration, influencing gene expression, and may provide mechanistic insights into functions associated with disease susceptibility. 3D genome simulation suggests a model of chromatin folding around chromosomal axes, where CTCF is involved in defining the interface between condensed and open compartments for structural regulation. Our 3D genome strategy thus provides unique insights in the topological mechanism of human variations and diseases.
Copyright © 2015 Elsevier Inc. All rights reserved.
Figures
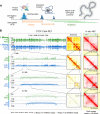
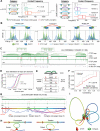
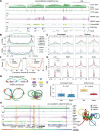
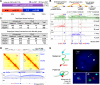
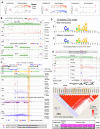
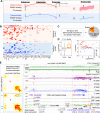
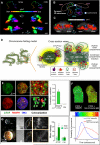
Similar articles
-
A role for CTCF and cohesin in subtelomere chromatin organization, TERRA transcription, and telomere end protection.EMBO J. 2012 Nov 5;31(21):4165-78. doi: 10.1038/emboj.2012.266. Epub 2012 Sep 25. EMBO J. 2012. PMID: 23010778 Free PMC article.
-
Topoisomerase II beta interacts with cohesin and CTCF at topological domain borders.Genome Biol. 2016 Aug 31;17(1):182. doi: 10.1186/s13059-016-1043-8. Genome Biol. 2016. PMID: 27582050 Free PMC article.
-
Genome-wide studies of CCCTC-binding factor (CTCF) and cohesin provide insight into chromatin structure and regulation.J Biol Chem. 2012 Sep 7;287(37):30906-13. doi: 10.1074/jbc.R111.324962. Epub 2012 Sep 5. J Biol Chem. 2012. PMID: 22952237 Free PMC article. Review.
-
Cohesin and CTCF differentially affect chromatin architecture and gene expression in human cells.Proc Natl Acad Sci U S A. 2014 Jan 21;111(3):996-1001. doi: 10.1073/pnas.1317788111. Epub 2013 Dec 13. Proc Natl Acad Sci U S A. 2014. PMID: 24335803 Free PMC article.
-
A tour of 3D genome with a focus on CTCF.Semin Cell Dev Biol. 2019 Jun;90:4-11. doi: 10.1016/j.semcdb.2018.07.020. Epub 2018 Jul 23. Semin Cell Dev Biol. 2019. PMID: 30031214 Review.
Cited by
-
ChIA-PIPE: A fully automated pipeline for comprehensive ChIA-PET data analysis and visualization.Sci Adv. 2020 Jul 10;6(28):eaay2078. doi: 10.1126/sciadv.aay2078. eCollection 2020 Jul. Sci Adv. 2020. PMID: 32832596 Free PMC article.
-
Epigenetic Vulnerability of Insulator CTCF Motifs at Parkinson's Disease-Associated Genes in Response to Neurotoxicant Rotenone.Front Genet. 2020 Jul 7;11:627. doi: 10.3389/fgene.2020.00627. eCollection 2020. Front Genet. 2020. PMID: 32774342 Free PMC article.
-
An integrated 3-Dimensional Genome Modeling Engine for data-driven simulation of spatial genome organization.Genome Res. 2016 Dec;26(12):1697-1709. doi: 10.1101/gr.205062.116. Epub 2016 Oct 27. Genome Res. 2016. PMID: 27789526 Free PMC article.
-
Defining and detecting links in chromosomes.Sci Rep. 2019 Aug 13;9(1):11753. doi: 10.1038/s41598-019-47999-4. Sci Rep. 2019. PMID: 31409805 Free PMC article.
-
Selfish: discovery of differential chromatin interactions via a self-similarity measure.Bioinformatics. 2019 Jul 15;35(14):i145-i153. doi: 10.1093/bioinformatics/btz362. Bioinformatics. 2019. PMID: 31510653 Free PMC article.
References
-
- Bickmore WA. The spatial organization of the human genome. Annu Rev Genomics Hum Genet. 2013;14:67–84. - PubMed
-
- Cremer M, Grasser F, Lanctot C, Muller S, Neusser M, Zinner R, Solovei I, Cremer T. Multicolor 3D fluorescence in situ hybridization for imaging interphase chromosomes. Methods Mol Biol. 2008;463:205–239. - PubMed
-
- Cullen KE, Kladde MP, Seyfred MA. Interaction between transcription regulatory regions of prolactin chromatin. Science. 1993;261:203–206. - PubMed
Publication types
MeSH terms
Substances
Associated data
- Actions
Grants and funding
LinkOut - more resources
Full Text Sources
Other Literature Sources
Molecular Biology Databases