Plasticity of corticospinal neural control after locomotor training in human spinal cord injury
- PMID: 22701805
- PMCID: PMC3373155
- DOI: 10.1155/2012/254948
Plasticity of corticospinal neural control after locomotor training in human spinal cord injury
Abstract
Spinal lesions substantially impair ambulation, occur generally in young and otherwise healthy individuals, and result in devastating effects on quality of life. Restoration of locomotion after damage to the spinal cord is challenging because axons of the damaged neurons do not regenerate spontaneously. Body-weight-supported treadmill training (BWSTT) is a therapeutic approach in which a person with a spinal cord injury (SCI) steps on a motorized treadmill while some body weight is removed through an upper body harness. BWSTT improves temporal gait parameters, muscle activation patterns, and clinical outcome measures in persons with SCI. These changes are likely the result of reorganization that occurs simultaneously in supraspinal and spinal cord neural circuits. This paper will focus on the cortical control of human locomotion and motor output, spinal reflex circuits, and spinal interneuronal circuits and how corticospinal control is reorganized after locomotor training in people with SCI. Based on neurophysiological studies, it is apparent that corticospinal plasticity is involved in restoration of locomotion after training. However, the neural mechanisms underlying restoration of lost voluntary motor function are not well understood and translational neuroscience research is needed so patient-orientated rehabilitation protocols to be developed.
Figures
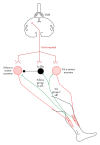
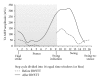
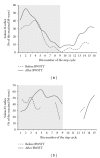
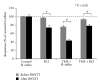
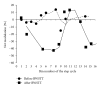
Similar articles
-
Robot-Applied Resistance Augments the Effects of Body Weight-Supported Treadmill Training on Stepping and Synaptic Plasticity in a Rodent Model of Spinal Cord Injury.Neurorehabil Neural Repair. 2017 Aug;31(8):746-757. doi: 10.1177/1545968317721016. Epub 2017 Jul 25. Neurorehabil Neural Repair. 2017. PMID: 28741434 Free PMC article.
-
Role of spared pathways in locomotor recovery after body-weight-supported treadmill training in contused rats.J Neurotrauma. 2011 Dec;28(12):2405-16. doi: 10.1089/neu.2010.1660. Epub 2011 Aug 8. J Neurotrauma. 2011. PMID: 21568686 Free PMC article.
-
Corticospinal Motor Circuit Plasticity After Spinal Cord Injury: Harnessing Neuroplasticity to Improve Functional Outcomes.Mol Neurobiol. 2021 Nov;58(11):5494-5516. doi: 10.1007/s12035-021-02484-w. Epub 2021 Aug 3. Mol Neurobiol. 2021. PMID: 34341881 Review.
-
A Single Bolus of Docosahexaenoic Acid Promotes Neuroplastic Changes in the Innervation of Spinal Cord Interneurons and Motor Neurons and Improves Functional Recovery after Spinal Cord Injury.J Neurosci. 2015 Sep 16;35(37):12733-52. doi: 10.1523/JNEUROSCI.0605-15.2015. J Neurosci. 2015. PMID: 26377463 Free PMC article.
-
Plasticity properties of CPG circuits in humans: impact on gait recovery.Brain Res Bull. 2009 Jan 15;78(1):22-5. doi: 10.1016/j.brainresbull.2008.02.030. Epub 2008 Mar 14. Brain Res Bull. 2009. PMID: 19070782 Review.
Cited by
-
Physiotherapy in the Recovery of Paraplegic Dogs without Nociception Due to Thoracolumbar Intervertebral Disc Extrusion Treated Surgically.Animals (Basel). 2024 Sep 12;14(18):2648. doi: 10.3390/ani14182648. Animals (Basel). 2024. PMID: 39335238 Free PMC article.
-
Repetitive Transcranial Magnetic Stimulation with Body Weight-supported Treadmill Training Enhances Independent Walking of Individuals with Chronic Incomplete Spinal Cord Injury: A Pilot Randomized Clinical Trial.Brain Topogr. 2024 Nov;37(6):1232-1241. doi: 10.1007/s10548-024-01072-0. Epub 2024 Aug 20. Brain Topogr. 2024. PMID: 39162868 Clinical Trial.
-
Approach to Small Animal Neurorehabilitation by Locomotor Training: An Update.Animals (Basel). 2022 Dec 18;12(24):3582. doi: 10.3390/ani12243582. Animals (Basel). 2022. PMID: 36552502 Free PMC article. Review.
-
Brain morphology changes after spinal cord injury: A voxel-based meta-analysis.Front Neurol. 2022 Sep 1;13:999375. doi: 10.3389/fneur.2022.999375. eCollection 2022. Front Neurol. 2022. PMID: 36119697 Free PMC article.
-
Pharmacological and non-pharmacological therapeutic interventions for the treatment of spinal cord injury-induced pain.Front Pain Res (Lausanne). 2022 Aug 24;3:991736. doi: 10.3389/fpain.2022.991736. eCollection 2022. Front Pain Res (Lausanne). 2022. PMID: 36093389 Free PMC article. Review.
References
-
- Raineteau O, Schwab ME. Plasticity of motor systems after incomplete spinal cord injury. Nature Reviews Neuroscience. 2001;2(4):263–273. - PubMed
-
- Smith HC, Savic G, Frankel HL, et al. Corticospinal function studied over time following incomplete spinal cord injury. Spinal Cord. 2000;38(5):292–300. - PubMed
-
- Bruehlmeier M, Dietz V, Leenders KL, Roelcke U, Missimer J, Curt A. How does the human brain deal with a spinal cord injury? European Journal of Neuroscience. 1998;10(12):3918–3922. - PubMed
-
- Curt A, Alkadhi H, Crelier GR, Boendermaker SH, Hepp-Reymond MC, Kollias SS. Changes of non-affected upper limb cortical representation in paraplegic patients as assessed by fMRI. Brain. 2002;125(11):2567–2578. - PubMed
Publication types
MeSH terms
LinkOut - more resources
Full Text Sources
Medical