Comparison of the transcription and replication strategies of marburg virus and Ebola virus by using artificial replication systems
- PMID: 9971816
- PMCID: PMC104478
- DOI: 10.1128/JVI.73.3.2333-2342.1999
Comparison of the transcription and replication strategies of marburg virus and Ebola virus by using artificial replication systems
Abstract
The members of the family Filoviridae, Marburg virus (MBGV) and Ebola virus (EBOV), are very similar in terms of morphology, genome organization, and protein composition. To compare the replication and transcription strategies of both viruses, an artificial replication system based on the vaccinia virus T7 expression system was established for EBOV. Specific transcription and replication of an artificial monocistronic minireplicon was demonstrated by reporter gene expression and detection of the transcribed and replicated RNA species. As it was shown previously for MBGV, three of the four EBOV nucleocapsid proteins, NP, VP35, and L, were essential and sufficient for replication. In contrast to MBGV, EBOV-specific transcription was dependent on the presence of the fourth nucleocapsid protein, VP30. When EBOV VP30 was replaced by MBGV VP30, EBOV-specific transcription was observed but with lower efficiency. Exchange of NP, VP35, and L between the two replication systems did not lead to detectable reporter gene expression. It was further observed that neither MBGV nor EBOV were able to replicate the heterologous minigenomes. A chimeric minigenome, however, containing the EBOV leader and the MBGV trailer was encapsidated, replicated, transcribed, and packaged by both viruses.
Figures
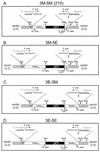
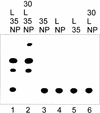
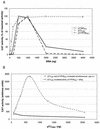
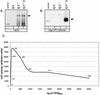
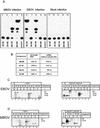
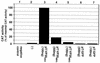
Similar articles
-
Three of the four nucleocapsid proteins of Marburg virus, NP, VP35, and L, are sufficient to mediate replication and transcription of Marburg virus-specific monocistronic minigenomes.J Virol. 1998 Nov;72(11):8756-64. doi: 10.1128/JVI.72.11.8756-8764.1998. J Virol. 1998. PMID: 9765419 Free PMC article.
-
A reconstituted replication and transcription system for Ebola virus Reston and comparison with Ebola virus Zaire.Virology. 2005 Feb 5;332(1):406-17. doi: 10.1016/j.virol.2004.11.018. Virology. 2005. PMID: 15661171
-
RNA Binding of Ebola Virus VP30 Is Essential for Activating Viral Transcription.J Virol. 2016 Jul 27;90(16):7481-7496. doi: 10.1128/JVI.00271-16. Print 2016 Aug 15. J Virol. 2016. PMID: 27279615 Free PMC article.
-
Evasion of interferon responses by Ebola and Marburg viruses.J Interferon Cytokine Res. 2009 Sep;29(9):511-20. doi: 10.1089/jir.2009.0076. J Interferon Cytokine Res. 2009. PMID: 19694547 Free PMC article. Review.
-
Virus and host interactions critical for filoviral RNA synthesis as therapeutic targets.Antiviral Res. 2019 Feb;162:90-100. doi: 10.1016/j.antiviral.2018.12.006. Epub 2018 Dec 11. Antiviral Res. 2019. PMID: 30550800 Free PMC article. Review.
Cited by
-
CAPG Is Required for Ebola Virus Infection by Controlling Virus Egress from Infected Cells.Viruses. 2022 Aug 28;14(9):1903. doi: 10.3390/v14091903. Viruses. 2022. PMID: 36146710 Free PMC article.
-
Ebola virus VP35 interaction with dynein LC8 regulates viral RNA synthesis.J Virol. 2015 May;89(9):5148-53. doi: 10.1128/JVI.03652-14. Epub 2015 Mar 4. J Virol. 2015. PMID: 25741013 Free PMC article.
-
Evidence for Viral mRNA Export from Ebola Virus Inclusion Bodies by the Nuclear RNA Export Factor NXF1.J Virol. 2022 Sep 28;96(18):e0090022. doi: 10.1128/jvi.00900-22. Epub 2022 Aug 30. J Virol. 2022. PMID: 36040180 Free PMC article.
-
Predictive and comparative analysis of Ebolavirus proteins.Cell Cycle. 2015;14(17):2785-97. doi: 10.1080/15384101.2015.1068472. Epub 2015 Jul 9. Cell Cycle. 2015. PMID: 26158395 Free PMC article.
-
Establishment and characterization of plasmid-driven minigenome rescue systems for Nipah virus: RNA polymerase I- and T7-catalyzed generation of functional paramyxoviral RNA.Virology. 2008 Jan 5;370(1):33-44. doi: 10.1016/j.virol.2007.08.008. Epub 2007 Sep 27. Virology. 2008. PMID: 17904180 Free PMC article.
References
-
- Banerjee A K, Barik S. Gene expression of vesicular stomatitis virus genome RNA. Virology. 1992;188:417–428. - PubMed
-
- Becker S, Mühlberger E. Co- and posttranslational modifications and functions of Marburg virus proteins. In: Klenk H-D, editor. Marburg and Ebola viruses. Berlin, Germany: Springer-Verlag; 1998. - PubMed
-
- Becker S, Rinne C, Hofsäss U, Klenk H-D, Mühlberger E. Interactions of Marburg virus nucleocapsid proteins. Virology. 1998;249:406–417. - PubMed
-
- Becker S, Klenk H-D, Mühlberger E. Intracellular transport and processing of the Marburg virus surface protein in vertebrate and insect cells. Virology. 1996;225:145–155. - PubMed
-
- Bowen E T, Lloyd G, Harris W J, Platt G S, Baskerville A, Vella E E. Viral haemorrhagic fever in southern Sudan and northern Zaire. Preliminary studies on the aetiological agent. Lancet. 1977;i:571–573. - PubMed
Publication types
MeSH terms
LinkOut - more resources
Full Text Sources
Other Literature Sources
Medical
Molecular Biology Databases
Research Materials
Miscellaneous