Functional coupling between replication and packaging of poliovirus replicon RNA
- PMID: 9847348
- PMCID: PMC103849
- DOI: 10.1128/JVI.73.1.427-435.1999
Functional coupling between replication and packaging of poliovirus replicon RNA
Abstract
Poliovirus RNA genomes that contained deletions in the capsid-coding regions were synthesized in monkey kidney cells that constitutively expressed T7 RNA polymerase. These replication-competent subgenomic RNAs, or replicons (G. Kaplan and V. R. Racaniello, J. Virol. 62:1687-1696, 1988), were encapsidated in trans by superinfecting polioviruses. When superinfecting poliovirus resistant to the antiviral compound guanidine was used, the RNA replication of the replicon RNAs could be inhibited independently of the RNA replication of the guanidine-resistant helper virus. Inhibiting the replication of the replicon RNA also profoundly inhibited its trans-encapsidation, even though the capsid proteins present in the cells could efficiently encapsidate the helper virus. The observed coupling between RNA replication and RNA packaging could account for the specificity of poliovirus RNA packaging in infected cells and the observed effects of mutations in the coding regions of nonstructural proteins on virion morphogenesis. It is suggested that this coupling results from direct interactions between the RNA replication machinery and the capsid proteins. The coupling of RNA packaging to RNA replication and of RNA replication to translation (J. E. Novak and K. Kirkegaard, Genes Dev. 8:1726-1737, 1994) could serve as mechanisms for late proofreading of poliovirus RNA, allowing only those RNA genomes capable of translating a full complement of functional RNA replication proteins to be propagated.
Figures
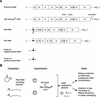
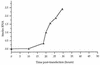
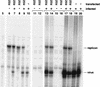
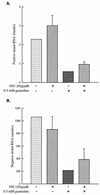
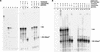
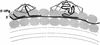
Similar articles
-
Encapsidation of poliovirus replicons encoding the complete human immunodeficiency virus type 1 gag gene by using a complementation system which provides the P1 capsid protein in trans.J Virol. 1995 Mar;69(3):1548-55. doi: 10.1128/JVI.69.3.1548-1555.1995. J Virol. 1995. PMID: 7853488 Free PMC article.
-
Construction and characterization of poliovirus subgenomic replicons.J Virol. 1988 May;62(5):1687-96. doi: 10.1128/JVI.62.5.1687-1696.1988. J Virol. 1988. PMID: 2833619 Free PMC article.
-
Inherent instability of poliovirus genomes containing two internal ribosome entry site (IRES) elements supports a role for the IRES in encapsidation.J Virol. 2000 Sep;74(18):8335-42. doi: 10.1128/jvi.74.18.8335-8342.2000. J Virol. 2000. PMID: 10954532 Free PMC article.
-
Expanding knowledge of P3 proteins in the poliovirus lifecycle.Future Microbiol. 2010 Jun;5(6):867-81. doi: 10.2217/fmb.10.40. Future Microbiol. 2010. PMID: 20521933 Free PMC article. Review.
-
Picornavirus morphogenesis.Microbiol Mol Biol Rev. 2014 Sep;78(3):418-37. doi: 10.1128/MMBR.00012-14. Microbiol Mol Biol Rev. 2014. PMID: 25184560 Free PMC article. Review.
Cited by
-
Rubella virus-like replicon particles: analysis of encapsidation determinants and non-structural roles of capsid protein in early post-entry replication.J Gen Virol. 2012 Mar;93(Pt 3):516-525. doi: 10.1099/vir.0.038984-0. Epub 2011 Nov 23. J Gen Virol. 2012. PMID: 22113006 Free PMC article.
-
Enteroviral proteases: structure, host interactions and pathogenicity.Rev Med Virol. 2016 Jul;26(4):251-67. doi: 10.1002/rmv.1883. Epub 2016 May 4. Rev Med Virol. 2016. PMID: 27145174 Free PMC article. Review.
-
Infection of human cells by dengue virus is modulated by different cell types and viral strains.J Virol. 2000 Sep;74(17):7814-23. doi: 10.1128/jvi.74.17.7814-7823.2000. J Virol. 2000. PMID: 10933688 Free PMC article.
-
Coxsackievirus expression of the murine secretory protein interleukin-4 induces increased synthesis of immunoglobulin G1 in mice.J Virol. 2000 Sep;74(17):7952-62. doi: 10.1128/jvi.74.17.7952-7962.2000. J Virol. 2000. PMID: 10933703 Free PMC article.
-
Translation of the flavivirus kunjin NS3 gene in cis but not its RNA sequence or secondary structure is essential for efficient RNA packaging.J Virol. 2006 Nov;80(22):11255-64. doi: 10.1128/JVI.01559-06. Epub 2006 Sep 13. J Virol. 2006. PMID: 16971441 Free PMC article.
References
-
- Ansardi D C, Porter D C, Anderson M J, Morrow C D. Poliovirus assembly and encapsidation of genomic RNA. Adv Virus Res. 1996;46:1–68. - PubMed
-
- Baltimore D. The replication of picornaviruses. In: Levy H B, editor. The biochemistry of viruses. New York, N.Y: Marcel Dekker, Inc.; 1969. pp. 101–176.
-
- Baltimore D, Girard M, Darnell J E. Aspects of the synthesis of poliovirus RNA and the formation of virus particles. Virology. 1966;29:179–189. - PubMed
Publication types
MeSH terms
Substances
Grants and funding
LinkOut - more resources
Full Text Sources
Other Literature Sources