The interaction between HIV-1 Tat and human cyclin T1 requires zinc and a critical cysteine residue that is not conserved in the murine CycT1 protein
- PMID: 9832504
- PMCID: PMC317238
- DOI: 10.1101/gad.12.22.3512
The interaction between HIV-1 Tat and human cyclin T1 requires zinc and a critical cysteine residue that is not conserved in the murine CycT1 protein
Abstract
HIV-1 Tat activates transcription through binding to human cyclin T1, a regulatory subunit of the TAK/P-TEFb CTD kinase complex. Here we show that the cyclin domain of hCycT1 is necessary and sufficient to interact with Tat and promote cooperative binding to TAR RNA in vitro, as well as mediate Tat transactivation in vivo. A Tat:TAR recognition motif (TRM) was identified at the carboxy-terminal edge of the cyclin domain, and we show that hCycT1 can interact simultaneously with Tat and CDK9 on TAR RNA in vitro. Alanine-scanning mutagenesis of the hCycT1 TRM identified residues that are critical for the interaction with Tat and others that are required specifically for binding of the complex to TAR RNA. Interestingly, we find that the interaction between Tat and hCycT1 requires zinc as well as essential cysteine residues in both proteins. Cloning and characterization of the murine CycT1 protein revealed that it lacks a critical cysteine residue (C261) and forms a weak, zinc-independent complex with HIV-1 Tat that greatly reduces binding to TAR RNA. A point mutation in mCycT1 (Y261C) restores high-affinity, zinc-dependent binding to Tat and TAR in vitro, and rescues Tat transactivation in vivo. Although overexpression of hCycT1 in NIH3T3 cells strongly enhances transcription from an integrated proviral promoter, we find that this fails to overcome all blocks to productive HIV-1 infection in murine cells.
Figures
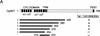
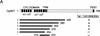
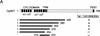
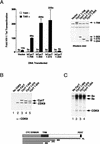
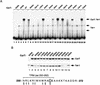
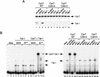
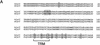
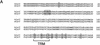
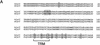
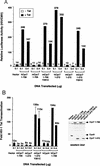
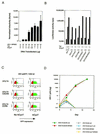
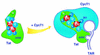
Similar articles
-
CDK9 autophosphorylation regulates high-affinity binding of the human immunodeficiency virus type 1 tat-P-TEFb complex to TAR RNA.Mol Cell Biol. 2000 Sep;20(18):6958-69. doi: 10.1128/MCB.20.18.6958-6969.2000. Mol Cell Biol. 2000. PMID: 10958691 Free PMC article.
-
Recruitment of a protein complex containing Tat and cyclin T1 to TAR governs the species specificity of HIV-1 Tat.EMBO J. 1998 Dec 1;17(23):7056-65. doi: 10.1093/emboj/17.23.7056. EMBO J. 1998. PMID: 9843510 Free PMC article.
-
A minimal chimera of human cyclin T1 and tat binds TAR and activates human immunodeficiency virus transcription in murine cells.J Virol. 2002 Dec;76(24):12934-9. doi: 10.1128/jvi.76.24.12934-12939.2002. J Virol. 2002. PMID: 12438619 Free PMC article.
-
Regulatory functions of Cdk9 and of cyclin T1 in HIV tat transactivation pathway gene expression.J Cell Biochem. 1999 Dec 1;75(3):357-68. J Cell Biochem. 1999. PMID: 10536359 Review.
-
Tackling Tat.J Mol Biol. 1999 Oct 22;293(2):235-54. doi: 10.1006/jmbi.1999.3060. J Mol Biol. 1999. PMID: 10550206 Review.
Cited by
-
The HIV-1 Tat Protein Is Monomethylated at Lysine 71 by the Lysine Methyltransferase KMT7.J Biol Chem. 2016 Jul 29;291(31):16240-8. doi: 10.1074/jbc.M116.735415. Epub 2016 May 27. J Biol Chem. 2016. PMID: 27235396 Free PMC article.
-
Transition step during assembly of HIV Tat:P-TEFb transcription complexes and transfer to TAR RNA.Mol Cell Biol. 2012 Dec;32(23):4780-93. doi: 10.1128/MCB.00206-12. Epub 2012 Sep 24. Mol Cell Biol. 2012. PMID: 23007159 Free PMC article.
-
TRIM5α and Species Tropism of HIV/SIV.Front Microbiol. 2012 Jan 24;3:13. doi: 10.3389/fmicb.2012.00013. eCollection 2012. Front Microbiol. 2012. PMID: 22291694 Free PMC article.
-
Identification of novel inhibitors of human immunodeficiency virus type 1 replication by in silico screening targeting cyclin T1/Tat interaction.Antimicrob Agents Chemother. 2013 Mar;57(3):1323-31. doi: 10.1128/AAC.01711-12. Epub 2012 Dec 28. Antimicrob Agents Chemother. 2013. PMID: 23274668 Free PMC article.
-
Human chromosome 2 carries a gene required for production of infectious human immunodeficiency virus type 1.J Virol. 2006 Apr;80(7):3406-15. doi: 10.1128/JVI.80.7.3406-3415.2006. J Virol. 2006. PMID: 16537608 Free PMC article.
References
-
- Bagby S, Kim S, Maldonado E, Tong K, Reinberg D, Ikura M. Solution structure of the carboxy-terminal core domain of human TFIIB: Similarity to cyclin A and interaction with TATA-binding protein. Cell. 1995;82:857–867. - PubMed
-
- Bartz S, Vodicka M. Production of high-titer human immunodeficiency virus type 1 pseudotyped with vesicular stomatitis virus glycoprotein. Methods. 1997;12:337–342. - PubMed
Publication types
MeSH terms
Substances
Associated data
- Actions
Grants and funding
LinkOut - more resources
Full Text Sources
Other Literature Sources
Molecular Biology Databases
Miscellaneous