Regulated targeting of BAX to mitochondria
- PMID: 9763432
- PMCID: PMC2132805
- DOI: 10.1083/jcb.143.1.207
Regulated targeting of BAX to mitochondria
Abstract
The proapoptotic protein BAX contains a single predicted transmembrane domain at its COOH terminus. In unstimulated cells, BAX is located in the cytosol and in peripheral association with intracellular membranes including mitochondria, but inserts into mitochondrial membranes after a death signal. This failure to insert into mitochondrial membrane in the absence of a death signal correlates with repression of the transmembrane signal-anchor function of BAX by the NH2-terminal domain. Targeting can be instated by deleting the domain or by replacing the BAX transmembrane segment with that of BCL-2. In stimulated cells, the contribution of the NH2 terminus of BAX correlates with further exposure of this domain after membrane insertion of the protein. The peptidyl caspase inhibitor zVAD-fmk partly blocks the stimulated mitochondrial membrane insertion of BAX in vivo, which is consistent with the ability of apoptotic cell extracts to support mitochondrial targeting of BAX in vitro, dependent on activation of caspase(s). Taken together, our results suggest that regulated targeting of BAX to mitochondria in response to a death signal is mediated by discrete domains within the BAX polypeptide. The contribution of one or more caspases may reflect an initiation and/or amplification of this regulated targeting.
Figures
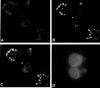
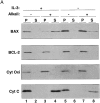
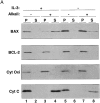
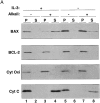
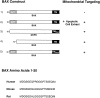
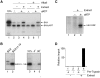
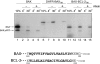
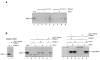
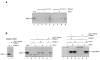
Similar articles
-
Bax membrane insertion during Fas(CD95)-induced apoptosis precedes cytochrome c release and is inhibited by Bcl-2.Oncogene. 1999 Oct 28;18(44):5991-9. doi: 10.1038/sj.onc.1203001. Oncogene. 1999. PMID: 10557088
-
BID-dependent and BID-independent pathways for BAX insertion into mitochondria.Cell Death Differ. 2000 Nov;7(11):1101-8. doi: 10.1038/sj.cdd.4400739. Cell Death Differ. 2000. PMID: 11139284
-
Targeting of Bcl-2 to the mitochondrial outer membrane by a COOH-terminal signal anchor sequence.J Biol Chem. 1993 Dec 5;268(34):25265-8. J Biol Chem. 1993. PMID: 8244956
-
vMIA, a viral inhibitor of apoptosis targeting mitochondria.Biochimie. 2002 Feb-Mar;84(2-3):177-85. doi: 10.1016/s0300-9084(02)01367-6. Biochimie. 2002. PMID: 12022948 Review.
-
Mechanisms of apoptosis in the heart.J Clin Immunol. 2003 Nov;23(6):447-59. doi: 10.1023/b:joci.0000010421.56035.60. J Clin Immunol. 2003. PMID: 15031632 Review.
Cited by
-
Cytosolic Bax: does it require binding proteins to keep its pro-apoptotic activity in check?J Biol Chem. 2012 Mar 16;287(12):9112-27. doi: 10.1074/jbc.M111.248906. Epub 2012 Jan 25. J Biol Chem. 2012. PMID: 22277657 Free PMC article.
-
Lentivirus-Mediated Overexpression of SIVA-1 Reverses Cisplatin Resistance in Gastric Cancer in vitro.Cell Biochem Biophys. 2020 Dec;78(4):455-463. doi: 10.1007/s12013-020-00929-y. Epub 2020 Jul 9. Cell Biochem Biophys. 2020. PMID: 32648086
-
Estrogen inhibits renal cell carcinoma cell progression through estrogen receptor-β activation.PLoS One. 2013;8(2):e56667. doi: 10.1371/journal.pone.0056667. Epub 2013 Feb 27. PLoS One. 2013. PMID: 23460808 Free PMC article.
-
Membrane remodeling induced by the dynamin-related protein Drp1 stimulates Bax oligomerization.Cell. 2010 Sep 17;142(6):889-901. doi: 10.1016/j.cell.2010.08.017. Cell. 2010. PMID: 20850011 Free PMC article.
-
A Bax-mediated mechanism for obatoclax-induced apoptosis of cholangiocarcinoma cells.Cancer Res. 2010 Mar 1;70(5):1960-9. doi: 10.1158/0008-5472.CAN-09-3535. Epub 2010 Feb 16. Cancer Res. 2010. PMID: 20160031 Free PMC article.
References
-
- Antonsson B, Conti F, Ciavatta A, Montessuit S, Lewis S, Martinou I, Bernasconi L, Bernard A, Mermod J-J, Mazzei G, et al. Inhibition of Bax channel-forming activity by BCL-2. Science. 1997;277:370–372. - PubMed
-
- Armstrong RC, Aja T, Xiang J, Gaur S, Krebs JF, Hoang K, Bai X, Korsmeyer SJ, Karanewsky DS, Fritz LC, Tomaselli KJ. Fas-induced activation of the cell death-related protease CPP32 is inhibited by BCL-2 and by ICE family protease inhibitors. J Biol Chem. 1996;271:16850–16855. - PubMed
-
- Boulakia CA, Chen G, Ng FWH, Teodoro JG, Branton PE, Nicholson DW, Poirier GG, Shore GC. BCL-2 and adenovirus E1B 19-kDa protein prevent E1A-induced processing of CPP32 and cleavage of poly(ADP-ribose) polymerase. Oncogene. 1996;12:529–535. - PubMed
-
- Cheng EH-Y, Levine B, Boise LH, Thompson CG, Hardwick JM. Bax-independent inhibition of apoptosis by BCL-XL . Nature. 1996;379:554–556. - PubMed
-
- Chinnaiyan AM, Orth K, O'Rourke K, Duan H, Poirier GG, Dixit VM. Molecular ordering of the cell death pathway. BCL-2 and BCL-XLfunction upstream of the CED-3-like apoptotic proteases. J Biol Chem. 1996;271:4573–4576. - PubMed
Publication types
MeSH terms
Substances
Grants and funding
LinkOut - more resources
Full Text Sources
Other Literature Sources
Research Materials