DNA-binding polarity of human replication protein A positions nucleases in nucleotide excision repair
- PMID: 9716411
- PMCID: PMC317078
- DOI: 10.1101/gad.12.16.2598
DNA-binding polarity of human replication protein A positions nucleases in nucleotide excision repair
Abstract
The human single-stranded DNA-binding replication A protein (RPA) is involved in various DNA-processing events. By comparing the affinity of hRPA for artificial DNA hairpin structures with 3'- or 5'-protruding single-stranded arms, we found that hRPA binds ssDNA with a defined polarity; a strong ssDNA interaction domain of hRPA is positioned at the 5' side of its binding region, a weak ssDNA-binding domain resides at the 3' side. Polarity appears crucial for positioning of the excision repair nucleases XPG and ERCC1-XPF on the DNA. With the 3'-oriented side of hRPA facing a duplex ssDNA junction, hRPA interacts with and stimulates ERCC1-XPF, whereas the 5'-oriented side of hRPA at a DNA junction allows stable binding of XPG to hRPA. Our data pinpoint hRPA to the undamaged strand during nucleotide excision repair. Polarity of hRPA on ssDNA is likely to contribute to the directionality of other hRPA-dependent processes as well.
Figures
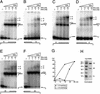
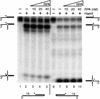
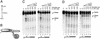
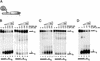
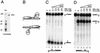
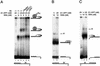
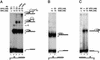
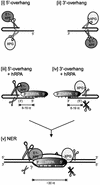
Similar articles
-
Replication protein A confers structure-specific endonuclease activities to the XPF-ERCC1 and XPG subunits of human DNA repair excision nuclease.J Biol Chem. 1996 May 10;271(19):11047-50. doi: 10.1074/jbc.271.19.11047. J Biol Chem. 1996. PMID: 8626644
-
Crystal structure and DNA binding functions of ERCC1, a subunit of the DNA structure-specific endonuclease XPF-ERCC1.Proc Natl Acad Sci U S A. 2005 Aug 9;102(32):11236-41. doi: 10.1073/pnas.0504341102. Epub 2005 Aug 2. Proc Natl Acad Sci U S A. 2005. PMID: 16076955 Free PMC article.
-
Single-stranded DNA Binding by the Helix-Hairpin-Helix Domain of XPF Protein Contributes to the Substrate Specificity of the ERCC1-XPF Protein Complex.J Biol Chem. 2017 Feb 17;292(7):2842-2853. doi: 10.1074/jbc.M116.747857. Epub 2016 Dec 27. J Biol Chem. 2017. PMID: 28028171 Free PMC article.
-
The ERCC1 and ERCC4 (XPF) genes and gene products.Gene. 2015 Sep 15;569(2):153-61. doi: 10.1016/j.gene.2015.06.026. Epub 2015 Jun 12. Gene. 2015. PMID: 26074087 Free PMC article. Review.
-
[Replication protein A as a major eukaryotic single-stranded DNA-binding protein and its role in DNA repair].Mol Biol (Mosk). 2016 Sep-Oct;50(5):735-750. doi: 10.7868/S0026898416030083. Mol Biol (Mosk). 2016. PMID: 27830676 Review. Russian.
Cited by
-
Fluorescent human RPA to track assembly dynamics on DNA.bioRxiv [Preprint]. 2023 Nov 23:2023.11.23.568455. doi: 10.1101/2023.11.23.568455. bioRxiv. 2023. Update in: Methods. 2024 Mar;223:95-105. doi: 10.1016/j.ymeth.2024.01.019 PMID: 38045304 Free PMC article. Updated. Preprint.
-
Biochemical analyses indicate that binding and cleavage specificities define the ordered processing of human Okazaki fragments by Dna2 and FEN1.Nucleic Acids Res. 2012 Aug;40(14):6774-86. doi: 10.1093/nar/gks388. Epub 2012 May 7. Nucleic Acids Res. 2012. PMID: 22570407 Free PMC article.
-
Replication protein A: directing traffic at the intersection of replication and repair.Front Biosci (Landmark Ed). 2010 Jun 1;15(3):883-900. doi: 10.2741/3652. Front Biosci (Landmark Ed). 2010. PMID: 20515732 Free PMC article. Review.
-
Other proteins interacting with XP proteins.Adv Exp Med Biol. 2008;637:103-12. doi: 10.1007/978-0-387-09599-8_11. Adv Exp Med Biol. 2008. PMID: 19181115 Free PMC article. Review. No abstract available.
-
OB-Folds and Genome Maintenance: Targeting Protein-DNA Interactions for Cancer Therapy.Cancers (Basel). 2021 Jul 3;13(13):3346. doi: 10.3390/cancers13133346. Cancers (Basel). 2021. PMID: 34283091 Free PMC article. Review.
References
Publication types
MeSH terms
Substances
LinkOut - more resources
Full Text Sources