Vascular endothelial growth factor-C (VEGF-C/VEGF-2) promotes angiogenesis in the setting of tissue ischemia
- PMID: 9708799
- PMCID: PMC1852989
- DOI: 10.1016/S0002-9440(10)65582-4
Vascular endothelial growth factor-C (VEGF-C/VEGF-2) promotes angiogenesis in the setting of tissue ischemia
Abstract
Recently, vascular endothelial growth factor-C (VEGF-C or VEGF-2) was described as a specific ligand for the endothelial receptor tyrosine kinases VEGFR-2 and VEGFR-3. In vivo data, limited to constitutive overexpression in transgenic mice, have been interpreted as evidence that the growth-promoting effects of VEGF-C are restricted to development of the lymphatic vasculature. The current studies were designed to test the hypothesis that constitutive expression of VEGF-C in adult animals promotes angiogenesis. In vitro, VEGF-C exhibited a dose-dependent mitogenic and chemotactic effect on endothelial cells, particularly for microvascular endothelial cells (72% and 95% potency, respectively, compared with VEGF-A/VEGF-1). VEGF-C stimulated release of nitric oxide from endothelial cells and increased vascular permeability in the Miles assay; the latter effect was attenuated by pretreatment with the nitric oxide synthase inhibitor N(omega)-nitro-L-arginine methyl ester. Both VEGFR-2 and VEGFR-3 receptors were shown to be expressed in human saphenous vein and internal mammary artery. The potential for VEGF-C to promote angiogenesis in vivo was then tested in a rabbit ischemic hindlimb model. Ten days after ligation of the external iliac artery, VEGF-C was administered as naked plasmid DNA (pcVEGF-C; 500 microg) from the polymer coating of an angioplasty balloon (n = 8 each) or as recombinant human protein (rhVEGF-C; 500 microg) by direct intra-arterial infusion. Physiological and anatomical assessments of angiogenesis 30 days later showed evidence of therapeutic angiogenesis for both pcVEGF-C and rhVEGF-C. Hindlimb blood pressure ratio (ischemic/normal) after pcVEGF-C increased to 0.83 +/- 0.03 after pcVEGF-C versus 0.59 +/- 0.04 (P < 0.005) in pGSVLacZ controls and to 0.76 +/- 0.04 after rhVEGF-C versus 0.58 +/- 0.03 (P < 0.01) in control rabbits receiving rabbit serum albumin. Doppler-derived iliac flow reserve was 2.7 +/- 0.1 versus 2.0 +/- 0.2 (P < 0.05) for pcVEGF-C versus LacZ controls and 2.9 +/- 0.3 versus 2.1 +/- 0.2 (P < 0.05) for rhVEGF-C versus albumin controls. Neovascularity was documented by angiography in vivo (angiographic scores: 0.85 +/- 0.05 versus 0.51 +/- 0.02 (P < 0.001) for plasmid DNA and 0.74 +/- 0.08 versus 0.53 +/- 0.03 (P < 0.05) for protein), and capillary density (per mm2) was measured at necropsy (252 +/- 12 versus 183 +/- 10 (P < 0.005) for plasmid DNA and 229 +/- 20 versus 164 +/- 20 (P < 0.05) for protein). In contrast to the results of gene targeting experiments, constitutive expression of VEGF-C in adult animals promotes angiogenesis in the setting of limb ischemia. VEGF-C and its receptors thus constitute an apparently redundant pathway for postnatal angiogenesis and may represent an alternative to VEGF-A for strategies of therapeutic angiogenesis in patients with limb and/or myocardial ischemia.
Figures
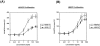
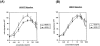
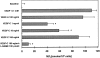
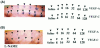
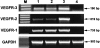
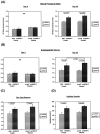
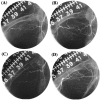
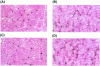
Similar articles
-
Vascular endothelial growth factor-B promotes in vivo angiogenesis.Circ Res. 2003 Jul 25;93(2):114-23. doi: 10.1161/01.RES.0000081594.21764.44. Epub 2003 Jun 12. Circ Res. 2003. PMID: 12805240
-
Vascular endothelial growth factor receptor-1 modulates vascular endothelial growth factor-mediated angiogenesis via nitric oxide.Am J Pathol. 2001 Sep;159(3):993-1008. doi: 10.1016/S0002-9440(10)61775-0. Am J Pathol. 2001. PMID: 11549592 Free PMC article.
-
VEGF-D is the strongest angiogenic and lymphangiogenic effector among VEGFs delivered into skeletal muscle via adenoviruses.Circ Res. 2003 May 30;92(10):1098-106. doi: 10.1161/01.RES.0000073584.46059.E3. Epub 2003 Apr 24. Circ Res. 2003. PMID: 12714562
-
A little VEGF goes a long way. Therapeutic angiogenesis by direct injection of vascular endothelial growth factor-encoding plasmid DNA.Circulation. 1996 Dec 15;94(12):3062-4. doi: 10.1161/01.cir.94.12.3062. Circulation. 1996. PMID: 8989106 Review.
-
[Regulation of the endothelial function and angiogenesis by vascular endothelial growth factor-A (VEGF-A].Z Kardiol. 2000 Mar;89(3):206-18. doi: 10.1007/s003920050472. Z Kardiol. 2000. PMID: 10798277 Review. German.
Cited by
-
Tissue-resident macrophages specifically express Lactotransferrin and Vegfc during ear pinna regeneration in spiny mice.Dev Cell. 2024 Feb 26;59(4):496-516.e6. doi: 10.1016/j.devcel.2023.12.017. Epub 2024 Jan 15. Dev Cell. 2024. PMID: 38228141
-
Quantification and cell-to-cell variation of vascular endothelial growth factor receptors.Exp Cell Res. 2011 Apr 15;317(7):955-65. doi: 10.1016/j.yexcr.2010.12.014. Epub 2010 Dec 23. Exp Cell Res. 2011. PMID: 21185287 Free PMC article.
-
Angiogenesis gene therapy to rescue ischaemic tissues: achievements and future directions.Br J Pharmacol. 2001 Aug;133(7):951-8. doi: 10.1038/sj.bjp.0704155. Br J Pharmacol. 2001. PMID: 11487503 Free PMC article. Review.
-
Establishment of bone marrow-derived endothelial cell lines from ts-SV40 T-antigen gene transgenic rats.Pharm Res. 2001 Jan;18(1):9-15. doi: 10.1023/a:1011062307374. Pharm Res. 2001. PMID: 11336358
-
Reversal of experimental diabetic neuropathy by VEGF gene transfer.J Clin Invest. 2001 May;107(9):1083-92. doi: 10.1172/JCI12188. J Clin Invest. 2001. PMID: 11342572 Free PMC article.
References
-
- Leung DW, Cachianes G, Kuang WJ, Goeddel DV, Ferrara N: Vascular endothelial growth factor is a secreted angiogenic mitogen. Science 1989, 246:1306-1309 - PubMed
-
- Senger DR, Galli SJ, Dvorak AM, Perruzzi CA, Harvey VS, Dvorak HF: Tumor cells secrete a vascular permeability factor that promotes accumulation of ascites fluid. Science 1983, 219:983-985 - PubMed
-
- Breier G, Albrecht U, Sterrer S, Risau W: Expression of vascular endothelial growth factor during embryonic angiogenesis and endothelial cell differentiation. Development 1992, 114:521-532 - PubMed
MeSH terms
Substances
LinkOut - more resources
Full Text Sources
Other Literature Sources
Miscellaneous