Localization-dependent translation requires a functional interaction between the 5' and 3' ends of oskar mRNA
- PMID: 9620852
- PMCID: PMC316867
- DOI: 10.1101/gad.12.11.1652
Localization-dependent translation requires a functional interaction between the 5' and 3' ends of oskar mRNA
Abstract
The precise restriction of proteins to specific domains within a cell plays an important role in early development and differentiation. An efficient way to localize and concentrate proteins is by localization of mRNA in a translationally repressed state, followed by activation of translation when the mRNA reaches its destination. A central issue is how localized mRNAs are derepressed. In this study we demonstrate that, when oskar mRNA reaches the posterior pole of the Drosophila oocyte, its translation is derepressed by an active process that requires a specific element in the 5' region of the mRNA. We demonstrate that this novel type of element is a translational derepressor element, whose functional interaction with the previously identified repressor region in the oskar 3' UTR is required for activation of oskar mRNA translation at the posterior pole. The derepressor element only functions at the posterior pole, suggesting that a locally restricted interaction between trans-acting factors and the derepressor element may be the link between mRNA localization and translational activation. We also show specific interaction of two proteins with the oskar mRNA 5' region; one of these also recognizes the 3' repressor element. We discuss the possible involvement of these factors as well as known genes in the process of localization-dependent translation.
Figures
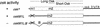
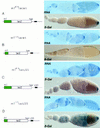
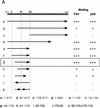
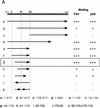
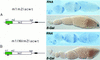
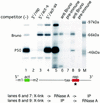
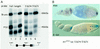
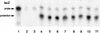
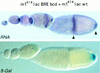
Similar articles
-
BREs mediate both repression and activation of oskar mRNA translation and act in trans.Dev Cell. 2010 Mar 16;18(3):496-502. doi: 10.1016/j.devcel.2009.12.021. Dev Cell. 2010. PMID: 20230756 Free PMC article.
-
Control of oskar mRNA translation by Bruno in a novel cell-free system from Drosophila ovaries.Development. 2000 Mar;127(5):1063-8. doi: 10.1242/dev.127.5.1063. Development. 2000. PMID: 10662645
-
Orb and a long poly(A) tail are required for efficient oskar translation at the posterior pole of the Drosophila oocyte.Development. 2003 Mar;130(5):835-43. doi: 10.1242/dev.00309. Development. 2003. PMID: 12538512
-
Localization, anchoring and translational control of oskar, gurken, bicoid and nanos mRNA during Drosophila oogenesis.Fly (Austin). 2009 Jan-Mar;3(1):15-28. doi: 10.4161/fly.3.1.7751. Epub 2009 Jan 2. Fly (Austin). 2009. PMID: 19182536 Review.
-
RNA localization and translational regulation during axis specification in the Drosophila oocyte.Int Rev Cytol. 2001;203:541-66. doi: 10.1016/s0074-7696(01)03016-9. Int Rev Cytol. 2001. PMID: 11131526 Review.
Cited by
-
An Interaction Network of RNA-Binding Proteins Involved in Drosophila Oogenesis.Mol Cell Proteomics. 2020 Sep;19(9):1485-1502. doi: 10.1074/mcp.RA119.001912. Epub 2020 Jun 17. Mol Cell Proteomics. 2020. PMID: 32554711 Free PMC article.
-
Interaction of Staufen1 with the 5' end of mRNA facilitates translation of these RNAs.Nucleic Acids Res. 2005 Aug 26;33(15):4797-812. doi: 10.1093/nar/gki794. Print 2005. Nucleic Acids Res. 2005. PMID: 16126845 Free PMC article.
-
BREs mediate both repression and activation of oskar mRNA translation and act in trans.Dev Cell. 2010 Mar 16;18(3):496-502. doi: 10.1016/j.devcel.2009.12.021. Dev Cell. 2010. PMID: 20230756 Free PMC article.
-
Assembly of the ribonucleoprotein complex containing the mRNA of the beta-subunit of the mitochondrial H+-ATP synthase requires the participation of two distal cis-acting elements and a complex set of cellular trans-acting proteins.Biochem J. 2002 Jul 15;365(Pt 2):417-28. doi: 10.1042/BJ20011726. Biochem J. 2002. PMID: 11952427 Free PMC article.
-
Region-specific activation of oskar mRNA translation by inhibition of Bruno-mediated repression.PLoS Genet. 2015 Feb 27;11(2):e1004992. doi: 10.1371/journal.pgen.1004992. eCollection 2015. PLoS Genet. 2015. PMID: 25723530 Free PMC article.
References
-
- Ausubel, F., R. Brent, R.E. Kingston, D.D. Moore, J.G. Seidman, J.A. Smith, and K. Struhl, eds. 1987–1998. Current protocols in molecular biology. Wiley & Sons, New York, NY.
-
- Castrillon D, Gonczy P, Alexander S, Rawson R, Eberhart CG, Viswanathan S, DiNardo S, Wasserman SA. Toward a molecular genetic analysis of spermatogenesis in Drosophila melanogaster: Characterization of male-sterile mutants generated by single P element mutagenesis. Genetics. 1993;135:489–505. - PMC - PubMed
-
- Clark I, Giniger E, Ruohola-Baker H, Jan LY, Jan YN. Transient posterior localization of a kinesin fusion protein reflects anteroposterior polarity of the Drosophila oocyte. Curr Biol. 1994;4:289–300. - PubMed
Publication types
MeSH terms
Substances
LinkOut - more resources
Full Text Sources
Other Literature Sources
Molecular Biology Databases