Endocytotic formation of vesicles and other membranous structures induced by Ca2+ and axolemmal injury
- PMID: 9592084
- PMCID: PMC6792792
- DOI: 10.1523/JNEUROSCI.18-11-04029.1998
Endocytotic formation of vesicles and other membranous structures induced by Ca2+ and axolemmal injury
Abstract
Vesicles and/or other membranous structures that form after axolemmal damage have recently been shown to repair (seal) the axolemma of various nerve axons. To determine the origin of such membranous structures, (1) we internally dialyzed isolated intact squid giant axons (GAs) and showed that elevation of intracellular Ca2+ >100 microM produced membranous structures similar to those in axons transected in Ca2+-containing physiological saline; (2) we exposed GA axoplasm to Ca2+-containing salines and observed that membranous structures did not form after removing the axolemma and glial sheath but did form in severed GAs after >99% of their axoplasm was removed by internal perfusion; (3) we examined transected GAs and crayfish medial giant axons (MGAs) with time-lapse confocal fluorescence microscopy and showed that many injury-induced vesicles formed by endocytosis of the axolemma; (4) we examined the cut ends of GAs and MGAs with electron microscopy and showed that most membranous structures were single-walled at short (5-15 min) post-transection times, whereas more were double- and multi-walled and of probable glial origin after longer (30-150 min) post-transection times; and (5) we examined differential interference contrast and confocal images and showed that large and small lesions evoked similar injury responses in which barriers to dye diffusion formed amid an accumulation of vesicles and other membranous structures. These and other data suggest that Ca2+ inflow at large or small axolemmal lesions induces various membranous structures (including endocytotic vesicles) of glial or axonal origin to form, accumulate, and interact with each other, preformed vesicles, and/or the axolemma to repair the axolemmal damage.
Figures
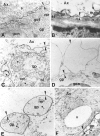
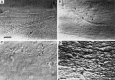
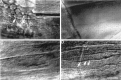
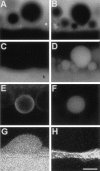
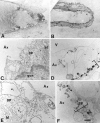
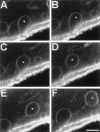
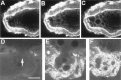
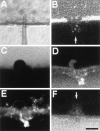
Similar articles
-
Axolemmal repair requires proteins that mediate synaptic vesicle fusion.J Neurobiol. 2000 Sep 15;44(4):382-91. doi: 10.1002/1097-4695(20000915)44:4<382::aid-neu2>3.0.co;2-q. J Neurobiol. 2000. PMID: 10945894
-
Delaminating myelin membranes help seal the cut ends of severed earthworm giant axons.J Neurobiol. 1997 Dec;33(7):945-60. doi: 10.1002/(sici)1097-4695(199712)33:7<945::aid-neu6>3.0.co;2-8. J Neurobiol. 1997. PMID: 9407015
-
Repair of plasmalemmal lesions by vesicles.Proc Natl Acad Sci U S A. 1997 Apr 29;94(9):4745-50. doi: 10.1073/pnas.94.9.4745. Proc Natl Acad Sci U S A. 1997. PMID: 9114062 Free PMC article.
-
Natural mechanisms and artificial PEG-induced mechanism that repair traumatic damage to the plasmalemma in eukaryotes.Curr Top Membr. 2019;84:129-167. doi: 10.1016/bs.ctm.2019.07.005. Epub 2019 Sep 26. Curr Top Membr. 2019. PMID: 31610860 Review.
-
A mechanistic analysis of nondisruptive axonal injury: a review.J Neurotrauma. 1997 Jul;14(7):419-40. doi: 10.1089/neu.1997.14.419. J Neurotrauma. 1997. PMID: 9257661 Review.
Cited by
-
Glia instruct axon regeneration via a ternary modulation of neuronal calcium channels in Drosophila.Nat Commun. 2023 Oct 14;14(1):6490. doi: 10.1038/s41467-023-42306-2. Nat Commun. 2023. PMID: 37838791 Free PMC article.
-
Repair of traumatic plasmalemmal damage to neurons and other eukaryotic cells.Neural Regen Res. 2016 Jul;11(7):1033-42. doi: 10.4103/1673-5374.187019. Neural Regen Res. 2016. PMID: 27630671 Free PMC article. Review.
-
Dysferlin and muscle membrane repair.Curr Opin Cell Biol. 2007 Aug;19(4):409-16. doi: 10.1016/j.ceb.2007.07.001. Epub 2007 Jul 26. Curr Opin Cell Biol. 2007. PMID: 17662592 Free PMC article. Review.
-
Temperature modulation reveals three distinct stages of Wallerian degeneration.J Neurosci. 1999 Jun 15;19(12):4718-26. doi: 10.1523/JNEUROSCI.19-12-04718.1999. J Neurosci. 1999. PMID: 10366605 Free PMC article.
-
Annexins and Membrane Repair Dysfunctions in Muscular Dystrophies.Int J Mol Sci. 2021 May 17;22(10):5276. doi: 10.3390/ijms22105276. Int J Mol Sci. 2021. PMID: 34067866 Free PMC article. Review.
References
-
- Ashery U, Penner R, Spira ME. Acceleration of membrane recycling by axotomy of cultured Aplysia neurons. Neuron. 1996;16:641–651. - PubMed
-
- Ballinger ML, Bittner GD. Ultrastructural studies of severed giant and other CNS axons in crayfish. Cell Tissue Res. 1980;208:123–133. - PubMed
-
- Ballinger ML, Blanchette AR, Krause TL, Smyers ME, Fishman HM, Bittner GD. Delaminating myelin membranes help seal the cut ends of severed earthworm giant axons. J Neurobiol. 1997;33:945–960. - PubMed
-
- Baumgold J, Terakawa S, Iwasa K, Gainer H. Membrane-associated cytoskeletal proteins in squid giant axons. J Neurochem. 1981;36:759–764. - PubMed
Publication types
MeSH terms
Substances
Grants and funding
LinkOut - more resources
Full Text Sources
Miscellaneous