The yeast HPR1 gene has a functional role in transcriptional elongation that uncovers a novel source of genome instability
- PMID: 9407037
- PMCID: PMC316820
- DOI: 10.1101/gad.11.24.3459
The yeast HPR1 gene has a functional role in transcriptional elongation that uncovers a novel source of genome instability
Abstract
The yeast HPR1 gene plays an important role in genome stability, as indicated by the observation that hpr1 mutants have high frequencies of DNA repeat recombination and chromosome loss. Here we report that HPR1 is required for transcriptional elongation. Transcription driven from constitutive and regulated yeast promoters cannot elongate through the bacterial lacZ coding region in hpr1Delta cells, but progresses efficiently through other sequences such as yeast PHO5. We show that HPR1 is not required for transcription activation and that the previously reported effects of hpr1Delta on the activation of different promoters is a consequence of the incapacity of hpr1Delta cells to elongate transcription through lacZ, used as reporter. Transcriptional defects are also observed in yeast DNA sequences of hpr1Delta cells in the presence of the transcription elongation inhibitor 6-azauracil. In all cases, the blockage of transcription elongation in hpr1Delta is associated with both the high frequency of deletions and the increase in plasmid instability that we report here. Therefore, in addition to the identification of a new element involved in transcriptional elongation, our work provides evidence for a new source of genomic instability.
Figures
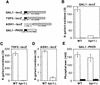
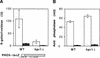
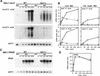
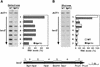
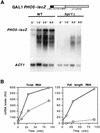
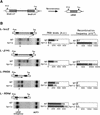
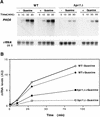
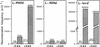
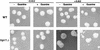
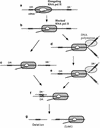
Similar articles
-
Recombination between DNA repeats in yeast hpr1delta cells is linked to transcription elongation.EMBO J. 1997 May 15;16(10):2826-35. doi: 10.1093/emboj/16.10.2826. EMBO J. 1997. PMID: 9184227 Free PMC article.
-
A novel yeast gene, THO2, is involved in RNA pol II transcription and provides new evidence for transcriptional elongation-associated recombination.EMBO J. 1998 Aug 17;17(16):4859-72. doi: 10.1093/emboj/17.16.4859. EMBO J. 1998. PMID: 9707445 Free PMC article.
-
A protein complex containing Tho2, Hpr1, Mft1 and a novel protein, Thp2, connects transcription elongation with mitotic recombination in Saccharomyces cerevisiae.EMBO J. 2000 Nov 1;19(21):5824-34. doi: 10.1093/emboj/19.21.5824. EMBO J. 2000. PMID: 11060033 Free PMC article.
-
Recombination proteins in yeast.Annu Rev Genet. 2004;38:233-71. doi: 10.1146/annurev.genet.38.072902.091500. Annu Rev Genet. 2004. PMID: 15568977 Review.
-
Transcription and chromatin converge: lessons from yeast genetics.Curr Opin Genet Dev. 2001 Apr;11(2):142-7. doi: 10.1016/s0959-437x(00)00171-4. Curr Opin Genet Dev. 2001. PMID: 11250136 Review.
Cited by
-
Homologous recombination is required for genome stability in the absence of DOG-1 in Caenorhabditis elegans.Genetics. 2006 Jun;173(2):697-708. doi: 10.1534/genetics.106.056879. Epub 2006 Mar 17. Genetics. 2006. PMID: 16547095 Free PMC article.
-
Transcription-Replication Collisions and Chromosome Fragility.Front Genet. 2021 Dec 10;12:804547. doi: 10.3389/fgene.2021.804547. eCollection 2021. Front Genet. 2021. PMID: 34956339 Free PMC article. Review.
-
The replication checkpoint protects fork stability by releasing transcribed genes from nuclear pores.Cell. 2011 Jul 22;146(2):233-46. doi: 10.1016/j.cell.2011.06.033. Cell. 2011. PMID: 21784245 Free PMC article.
-
Yeast genetic analysis reveals the involvement of chromatin reassembly factors in repressing HIV-1 basal transcription.PLoS Genet. 2009 Jan;5(1):e1000339. doi: 10.1371/journal.pgen.1000339. Epub 2009 Jan 16. PLoS Genet. 2009. PMID: 19148280 Free PMC article.
-
The contribution of co-transcriptional RNA:DNA hybrid structures to DNA damage and genome instability.DNA Repair (Amst). 2014 Jul;19:84-94. doi: 10.1016/j.dnarep.2014.03.023. Epub 2014 Apr 18. DNA Repair (Amst). 2014. PMID: 24746923 Free PMC article. Review.
References
-
- Aso T, Lane WS, Conaway JW, Conaway RC. Elongin (SIII): A multisubunit regulator of elongation by RNA polymerase II. Science. 1995;269:1439–1443. - PubMed
Publication types
MeSH terms
Substances
LinkOut - more resources
Full Text Sources
Molecular Biology Databases