Rescue of osteoclast function by transgenic expression of kinase-deficient Src in src-/- mutant mice
- PMID: 9353253
- PMCID: PMC316651
- DOI: 10.1101/gad.11.21.2835
Rescue of osteoclast function by transgenic expression of kinase-deficient Src in src-/- mutant mice
Abstract
The Src tyrosine kinase has been implicated in a wide variety of signal transduction pathways, yet despite the nearly ubiquitous expression of c-src, src-/- mice show only one major phenotype-osteopetrosis caused by an intrinsic defect in osteoclasts, the cells responsible for resorbing bone. To explore further the role of Src both in osteoclasts and other cell types, we have generated transgenic mice that express the wild-type and mutated versions of the chicken c-src proto-oncogene from the promoter of tartrate resistant acid phosphatase (TRAP), a gene that is expressed highly in osteoclasts. We demonstrate here that expression of a wild-type transgene in only a limited number of tissues can fully rescue the src-/- phenotype. Surprisingly, expression of kinase-defective alleles of c-src also reduces osteopetrosis in src-/- animals and partially rescues a defect in cytoskeletal organization observed in src-/- osteoclasts. These results suggest that there are essential kinase-independent functions for Src in vivo. Biochemical examination of osteoclasts from these mice suggest that Src may function in part by recruiting or activating other tyrosine kinases.
Figures
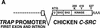
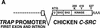
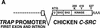
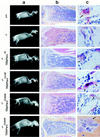
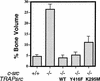
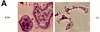
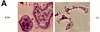
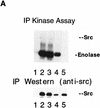
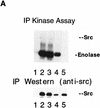
Similar articles
-
Targeted disruption of leucine-rich repeat kinase 1 but not leucine-rich repeat kinase 2 in mice causes severe osteopetrosis.J Bone Miner Res. 2013 Sep;28(9):1962-74. doi: 10.1002/jbmr.1935. J Bone Miner Res. 2013. PMID: 23526378 Free PMC article.
-
The Src signaling pathway regulates osteoclast lysosomal enzyme secretion and is rapidly modulated by estrogen.J Bone Miner Res. 2001 Jun;16(6):1028-36. doi: 10.1359/jbmr.2001.16.6.1028. J Bone Miner Res. 2001. PMID: 11393779
-
Extensive clear zone and defective ruffled border formation in osteoclasts of osteopetrotic (ia/ia) rats: implications for secretory function.Exp Cell Res. 1999 Sep 15;251(2):477-91. doi: 10.1006/excr.1999.4585. Exp Cell Res. 1999. PMID: 10471332
-
Role of c-Src in cellular events associated with colony-stimulating factor-1-induced spreading in osteoclasts.Mol Reprod Dev. 1997 Jan;46(1):104-8. doi: 10.1002/(SICI)1098-2795(199701)46:1<104::AID-MRD16>3.0.CO;2-2. Mol Reprod Dev. 1997. PMID: 8981371 Review.
-
Tartrate-resistant acid phosphatase knockout mice.J Bone Miner Res. 2003 Oct;18(10):1905-7. doi: 10.1359/jbmr.2003.18.10.1905. J Bone Miner Res. 2003. PMID: 14584904 Review.
Cited by
-
c-Src links a RANK/αvβ3 integrin complex to the osteoclast cytoskeleton.Mol Cell Biol. 2012 Jul;32(14):2943-53. doi: 10.1128/MCB.00077-12. Epub 2012 May 21. Mol Cell Biol. 2012. PMID: 22615494 Free PMC article.
-
Src inhibitors in the treatment of metastatic bone disease: rationale and clinical data.Clin Investig (Lond). 2011 Dec 1;1(12):1695-1706. doi: 10.4155/cli.11.150. Clin Investig (Lond). 2011. PMID: 22384312 Free PMC article.
-
Critical roles of c-Jun signaling in regulation of NFAT family and RANKL-regulated osteoclast differentiation.J Clin Invest. 2004 Aug;114(4):475-84. doi: 10.1172/JCI19657. J Clin Invest. 2004. PMID: 15314684 Free PMC article.
-
Dasatinib inhibits both osteoclast activation and prostate cancer PC-3-cell-induced osteoclast formation.Cancer Biol Ther. 2009 Nov;8(22):2153-9. doi: 10.4161/cbt.8.22.9770. Epub 2009 Nov 8. Cancer Biol Ther. 2009. PMID: 19855158 Free PMC article.
-
Elevated c-Src is linked to altered cell-matrix adhesion rather than proliferation in KM12C human colorectal cancer cells.Br J Cancer. 2002 Nov 4;87(10):1128-35. doi: 10.1038/sj.bjc.6600594. Br J Cancer. 2002. PMID: 12402152 Free PMC article.
References
-
- Baron R, Revesloot J-H, Neff L, Chakraborty M, Chatterjee D, Lomri A, Horne W. Cellular and molecular biology of bone. San Diego: Academic Press; 1993. Cellular and molecular biology of the osteoclast; pp. 445–495. California.
-
- Boyce BF, Wright K, Reddy SV, Koop BA, Story B, Devlin R, Leach RJ, Roodman GD, Windle JJ. Targeting simian virus 40 T antigen to the osteoclast in transgenic mice causes osteoclast tumors and transformation and apoptosis of osteoclasts. Endocrinology. 1995;136:5751–5759. - PubMed
-
- Boyce BF, Aufdemorte TB, Garrett IR, Yates AJP, Mundy GR. Effects of interleukin-1 on bone turnover in normal mice. Endocrinology. 1989;125:1142–1150. - PubMed
-
- Broome, M. 1996. PhD thesis. University of California, San Diego, CA.
Publication types
MeSH terms
Substances
Grants and funding
LinkOut - more resources
Full Text Sources
Other Literature Sources
Molecular Biology Databases
Miscellaneous