AU-rich elements target small nuclear RNAs as well as mRNAs for rapid degradation
- PMID: 9334320
- PMCID: PMC316563
- DOI: 10.1101/gad.11.19.2557
AU-rich elements target small nuclear RNAs as well as mRNAs for rapid degradation
Abstract
AU-rich elements (AREs, usually containing repeated copies of AUUUA), when present in the 3'-untranslated regions (UTRs) of many mammalian mRNAs, confer instability on their host RNA molecules. The viral small nuclear RNA (snRNA) Herpesvirus saimiri U RNA 1 (HSUR 1) also contains an AUUUA-rich sequence. Here, we report that this ARE induces rapid degradation of HSUR 1 itself and of other snRNAs including HSUR 2 and cellular U1. Mutational analyses of the viral ARE establish that sequence requirements for mRNA and snRNA decay are the same, suggesting a similar mechanism. Moreover, the in vivo degradation activity of mutant AREs correlates with their in vitro binding to the HuR protein, implicated previously as a component of the mRNA degradation machinery. Our results suggest that ARE-mediated instability can be uncoupled from both ongoing translation and deadenylation of the target RNA.
Figures
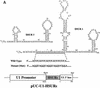
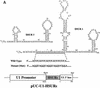
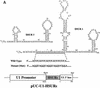
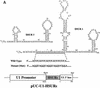
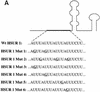
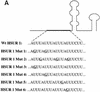
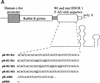
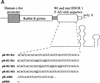
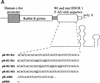
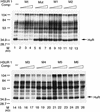
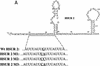
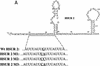
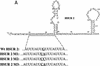
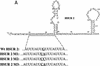
Similar articles
-
The Herpesvirus saimiri small nuclear RNAs recruit AU-rich element-binding proteins but do not alter host AU-rich element-containing mRNA levels in virally transformed T cells.Mol Cell Biol. 2004 May;24(10):4522-33. doi: 10.1128/MCB.24.10.4522-4533.2004. Mol Cell Biol. 2004. PMID: 15121869 Free PMC article.
-
Herpesvirus saimiri small RNA and interleukin-4 mRNA AUUUA repeats compete for sequence-specific factors including a novel 70K protein.J Gen Virol. 1994 Sep;75 ( Pt 9):2293-301. doi: 10.1099/0022-1317-75-9-2293. J Gen Virol. 1994. PMID: 8077927
-
Viral small nuclear ribonucleoproteins bind a protein implicated in messenger RNA destabilization.Proc Natl Acad Sci U S A. 1992 Feb 15;89(4):1296-300. doi: 10.1073/pnas.89.4.1296. Proc Natl Acad Sci U S A. 1992. PMID: 1311093 Free PMC article.
-
HuR and mRNA stability.Cell Mol Life Sci. 2001 Feb;58(2):266-77. doi: 10.1007/PL00000854. Cell Mol Life Sci. 2001. PMID: 11289308 Free PMC article. Review.
-
Novel roles for Sm-class RNAs in the regulation of gene expression.RNA Biol. 2018;15(7):856-862. doi: 10.1080/15476286.2018.1467176. Epub 2018 Jul 9. RNA Biol. 2018. PMID: 29895222 Free PMC article. Review.
Cited by
-
Mechanisms of deadenylation-dependent decay.Wiley Interdiscip Rev RNA. 2011 Mar-Apr;2(2):167-83. doi: 10.1002/wrna.40. Epub 2010 Sep 15. Wiley Interdiscip Rev RNA. 2011. PMID: 21957004 Free PMC article. Review.
-
Identifying mRNA subsets in messenger ribonucleoprotein complexes by using cDNA arrays.Proc Natl Acad Sci U S A. 2000 Dec 19;97(26):14085-90. doi: 10.1073/pnas.97.26.14085. Proc Natl Acad Sci U S A. 2000. PMID: 11121017 Free PMC article.
-
Inflammation: cytokines and RNA-based regulation.Wiley Interdiscip Rev RNA. 2010 Jul-Aug;1(1):60-80. doi: 10.1002/wrna.1. Epub 2010 May 6. Wiley Interdiscip Rev RNA. 2010. PMID: 21956907 Free PMC article. Review.
-
MRNA stability and the control of gene expression: implications for human disease.Neurochem Res. 2002 Oct;27(10):957-80. doi: 10.1023/a:1020992418511. Neurochem Res. 2002. PMID: 12462398 Review.
-
The Herpesvirus saimiri small nuclear RNAs recruit AU-rich element-binding proteins but do not alter host AU-rich element-containing mRNA levels in virally transformed T cells.Mol Cell Biol. 2004 May;24(10):4522-33. doi: 10.1128/MCB.24.10.4522-4533.2004. Mol Cell Biol. 2004. PMID: 15121869 Free PMC article.
References
-
- Belasco J, Brawerman G. Control of messenger RNA stability. San Diego, CA: Academic Press; 1993.
-
- Biesinger B, Trimble JJ, Desrosiers RC, Fleckenstein B. The divergence between two oncogenic herpesvirus saimiri strains in a genomic region related to the transforming phenotype. Virology. 1990;176:505–514. - PubMed
Publication types
MeSH terms
Substances
Grants and funding
LinkOut - more resources
Full Text Sources
Other Literature Sources
Miscellaneous