The membrane protein alkaline phosphatase is delivered to the vacuole by a route that is distinct from the VPS-dependent pathway
- PMID: 9245784
- PMCID: PMC2141640
- DOI: 10.1083/jcb.138.3.531
The membrane protein alkaline phosphatase is delivered to the vacuole by a route that is distinct from the VPS-dependent pathway
Abstract
Membrane trafficking intermediates involved in the transport of proteins between the TGN and the lysosome-like vacuole in the yeast Saccharomyces cerevisiae can be accumulated in various vps mutants. Loss of function of Vps45p, an Sec1p-like protein required for the fusion of Golgi-derived transport vesicles with the prevacuolar/endosomal compartment (PVC), results in an accumulation of post-Golgi transport vesicles. Similarly, loss of VPS27 function results in an accumulation of the PVC since this gene is required for traffic out of this compartment. The vacuolar ATPase subunit Vph1p transits to the vacuole in the Golgi-derived transport vesicles, as defined by mutations in VPS45, and through the PVC, as defined by mutations in VPS27. In this study we demonstrate that, whereas VPS45 and VPS27 are required for the vacuolar delivery of several membrane proteins, the vacuolar membrane protein alkaline phosphatase (ALP) reaches its final destination without the function of these two genes. Using a series of ALP derivatives, we find that the information to specify the entry of ALP into this alternative pathway to the vacuole is contained within its cytosolic tail, in the 13 residues adjacent to the transmembrane domain, and loss of this sorting determinant results in a protein that follows the VPS-dependent pathway to the vacuole. Using a combination of immunofluorescence localization and pulse/chase immunoprecipitation analysis, we demonstrate that, in addition to ALP, the vacuolar syntaxin Vam3p also follows this VPS45/27-independent pathway to the vacuole. In addition, the function of Vam3p is required for membrane traffic along the VPS-independent pathway.
Figures
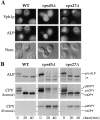
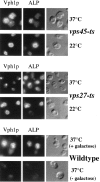
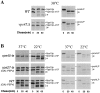
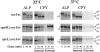
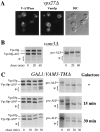
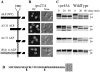
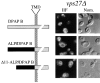
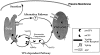
Similar articles
-
Traffic into the prevacuolar/endosomal compartment of Saccharomyces cerevisiae: a VPS45-dependent intracellular route and a VPS45-independent, endocytic route.Eur J Cell Biol. 1998 May;76(1):43-52. doi: 10.1016/S0171-9335(98)80016-2. Eur J Cell Biol. 1998. PMID: 9650782
-
Retrieval of resident late-Golgi membrane proteins from the prevacuolar compartment of Saccharomyces cerevisiae is dependent on the function of Grd19p.J Cell Biol. 1998 Feb 9;140(3):577-90. doi: 10.1083/jcb.140.3.577. J Cell Biol. 1998. PMID: 9456318 Free PMC article.
-
Novel Golgi to vacuole delivery pathway in yeast: identification of a sorting determinant and required transport component.EMBO J. 1997 May 15;16(10):2769-82. doi: 10.1093/emboj/16.10.2769. EMBO J. 1997. PMID: 9184222 Free PMC article.
-
Multiple sorting pathways between the late Golgi and the vacuole in yeast.Biochim Biophys Acta. 1998 Aug 14;1404(1-2):211-30. doi: 10.1016/s0167-4889(98)00058-5. Biochim Biophys Acta. 1998. PMID: 9714809 Review.
-
Receptor-mediated protein sorting to the vacuole in yeast: roles for a protein kinase, a lipid kinase and GTP-binding proteins.Annu Rev Cell Dev Biol. 1995;11:1-33. doi: 10.1146/annurev.cb.11.110195.000245. Annu Rev Cell Dev Biol. 1995. PMID: 8689553 Review.
Cited by
-
Cellular environment is important in controlling V-ATPase dissociation and its dependence on activity.J Biol Chem. 2007 Aug 24;282(34):24743-51. doi: 10.1074/jbc.M700663200. Epub 2007 Jun 12. J Biol Chem. 2007. PMID: 17565997 Free PMC article.
-
A novel mechanism for localizing membrane proteins to yeast trans-Golgi network requires function of synaptojanin-like protein.Mol Biol Cell. 2001 Oct;12(10):3175-90. doi: 10.1091/mbc.12.10.3175. Mol Biol Cell. 2001. PMID: 11598201 Free PMC article.
-
Isolation and characterization of Aspergillus oryzae vacuolar protein sorting mutants.Appl Environ Microbiol. 2005 Aug;71(8):4856-61. doi: 10.1128/AEM.71.8.4856-4861.2005. Appl Environ Microbiol. 2005. PMID: 16085884 Free PMC article.
-
The vacuolar kinase Yck3 maintains organelle fragmentation by regulating the HOPS tethering complex.J Cell Biol. 2005 Jan 31;168(3):401-14. doi: 10.1083/jcb.200407141. J Cell Biol. 2005. PMID: 15684030 Free PMC article.
-
Global analysis of yeast endosomal transport identifies the vps55/68 sorting complex.Mol Biol Cell. 2008 Apr;19(4):1282-94. doi: 10.1091/mbc.e07-07-0659. Epub 2008 Jan 23. Mol Biol Cell. 2008. PMID: 18216282 Free PMC article.
References
Publication types
MeSH terms
Substances
Grants and funding
LinkOut - more resources
Full Text Sources
Other Literature Sources
Molecular Biology Databases
Miscellaneous