Regulated production of mineralization-competent matrix vesicles in hypertrophic chondrocytes
- PMID: 9166414
- PMCID: PMC2136219
- DOI: 10.1083/jcb.137.5.1149
Regulated production of mineralization-competent matrix vesicles in hypertrophic chondrocytes
Abstract
Matrix vesicles have a critical role in the initiation of mineral deposition in skeletal tissues, but the ways in which they exert this key function remain poorly understood. This issue is made even more intriguing by the fact that matrix vesicles are also present in nonmineralizing tissues. Thus, we tested the novel hypothesis that matrix vesicles produced and released by mineralizing cells are structurally and functionally different from those released by nonmineralizing cells. To test this hypothesis, we made use of cultures of chick embryonic hypertrophic chondrocytes in which mineralization was triggered by treatment with vitamin C and phosphate. Ultrastructural analysis revealed that both control nonmineralizing and vitamin C/phosphatetreated mineralizing chondrocytes produced and released matrix vesicles that exhibited similar round shape, smooth contour, and average size. However, unlike control vesicles, those produced by mineralizing chondrocytes had very strong alkaline phosphatase activity and contained annexin V, a membrane-associated protein known to mediate Ca2+ influx into matrix vesicles. Strikingly, these vesicles also formed numerous apatite-like crystals upon incubation with synthetic cartilage lymph, while control vesicles failed to do so. Northern blot and immunohistochemical analyses showed that the production and release of annexin V-rich matrix vesicles by mineralizing chondrocytes were accompanied by a marked increase in annexin V expression and, interestingly, were followed by increased expression of type I collagen. Studies on embryonic cartilages demonstrated a similar sequence of phenotypic changes during the mineralization process in vivo. Thus, chondrocytes located in the hypertrophic zone of chick embryo tibial growth plate were characterized by strong annexin V expression, and those located at the chondro-osseous mineralizing border exhibited expression of both annexin V and type I collagen. These findings reveal that hypertrophic chondrocytes can qualitatively modulate their production of matrix vesicles and only when induced to initiate mineralization, will release mineralization-competent matrix vesicles rich in annexin V and alkaline phosphatase. The occurrence of type I collagen in concert with cartilage matrix calcification suggests that the protein may facilitate crystal growth after rupture of the matrix vesicle membrane; it may also offer a smooth transition from mineralized type II/type X collagen-rich cartilage matrix to type I collagen-rich bone matrix.
Figures
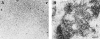
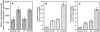
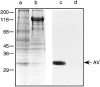
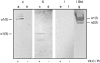
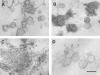
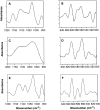
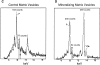
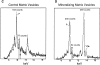
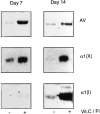
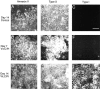
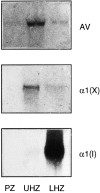
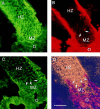
Similar articles
-
Retinoic acid stimulates annexin-mediated growth plate chondrocyte mineralization.J Cell Biol. 2002 Jun 10;157(6):1061-9. doi: 10.1083/jcb.200203014. Epub 2002 Jun 3. J Cell Biol. 2002. PMID: 12045186 Free PMC article.
-
Remodelling of collagen types I, II and X and calcification of human fetal cartilage.Bone Miner. 1992 Aug;18(2):107-17. doi: 10.1016/0169-6009(92)90851-4. Bone Miner. 1992. PMID: 1525593
-
Primary culture of rat growth plate chondrocytes: an in vitro model of growth plate histotype, matrix vesicle biogenesis and mineralization.Bone. 2004 Jun;34(6):961-70. doi: 10.1016/j.bone.2004.02.010. Bone. 2004. PMID: 15193542
-
Molecular biology of matrix vesicles.Clin Orthop Relat Res. 1995 May;(314):266-80. Clin Orthop Relat Res. 1995. PMID: 7634645 Review.
-
Matrix vesicles and calcification.Curr Rheumatol Rep. 2003 Jun;5(3):222-6. doi: 10.1007/s11926-003-0071-z. Curr Rheumatol Rep. 2003. PMID: 12744815 Review.
Cited by
-
Histopathological assessment of calcification and inflammation of calcific aortic valves from patients with and without diabetes mellitus.Histol Histopathol. 2017 Mar;32(3):293-306. doi: 10.14670/HH-11-797. Epub 2016 Jun 29. Histol Histopathol. 2017. PMID: 27353274 Free PMC article.
-
Insulin-like growth factor-I is essential for embryonic bone development.Endocrinology. 2006 Oct;147(10):4753-61. doi: 10.1210/en.2006-0196. Epub 2006 Jul 20. Endocrinology. 2006. PMID: 16857753 Free PMC article.
-
Dentin phosphoprotein binds annexin 2 and is involved in calcium transport in rat kidney ureteric bud cells.J Biol Chem. 2013 May 3;288(18):13036-45. doi: 10.1074/jbc.M112.389627. Epub 2013 Mar 22. J Biol Chem. 2013. PMID: 23525114 Free PMC article.
-
Do Media Extracellular Vesicles and Extracellular Vesicles Bound to the Extracellular Matrix Represent Distinct Types of Vesicles?Biomolecules. 2023 Dec 28;14(1):42. doi: 10.3390/biom14010042. Biomolecules. 2023. PMID: 38254642 Free PMC article. Review.
-
New developments in the pathogenesis of articular cartilage calcification.Curr Rheumatol Rep. 1999 Dec;1(2):121-7. doi: 10.1007/s11926-999-0008-2. Curr Rheumatol Rep. 1999. PMID: 11123025 Review.
References
-
- Adams SL, Pallante KM, Niu Z, Leboy PS, Golden EB, Pacifici M. Rapid induction of type X collagen gene expression in cultured chick vertebral chondrocytes. Exp Cell Res. 1991;193:190–197. - PubMed
-
- Ali SY. Analysis of matrix vesicles and their role in the calcification of epiphyseal cartilage. Fed Proc. 1976;35:135–142. - PubMed
-
- Ali SY. New knowledge of osteoarthritis. J Clin Pathol (Lond) 1992;178:205–213.
-
- Ames BN. Assay of inorganic phosphate, total phosphate and phosphatases. Methods Enzymol. 1966;8:115–118.
Publication types
MeSH terms
Substances
Grants and funding
LinkOut - more resources
Full Text Sources
Miscellaneous