Inactivation of two Dictyostelium discoideum genes, DdPIK1 and DdPIK2, encoding proteins related to mammalian phosphatidylinositide 3-kinases, results in defects in endocytosis, lysosome to postlysosome transport, and actin cytoskeleton organization
- PMID: 9087443
- PMCID: PMC2132510
- DOI: 10.1083/jcb.136.6.1271
Inactivation of two Dictyostelium discoideum genes, DdPIK1 and DdPIK2, encoding proteins related to mammalian phosphatidylinositide 3-kinases, results in defects in endocytosis, lysosome to postlysosome transport, and actin cytoskeleton organization
Abstract
Phosphatidylinositide 3-kinases (PI3-kinases) have been implicated in controlling cell proliferation, actin cytoskeleton organization, and the regulation of vesicle trafficking between intracellular organelles. There are at least three genes in Dictyostelium discoideum. DdPIK1, DdPIK2, and DdPIK3, encoding proteins most closely related to the mammalian 110-kD PI-3 kinase in amino acid sequence within the kinase domain. A mutant disrupted in DdPIK1 and DdPIK2 (delta ddpik1/ddpik2) grows slowly in liquid medium. Using FITC-dextran (FD) as a fluid phase marker, we determined that the mutant strain was impaired in pinocytosis but normal in phagocytosis of beads or bacteria. Microscopic and biochemical approaches indicated that the transport rate of fluid-phase from acidic lysosomes to non-acidic postlysosomal vacuoles was reduced in mutant cells resulting in a reduction in efflux of fluid phase. Mutant cells were also almost completely devoid of large postlysosomal vacuoles as determined by transmission EM. However, delta ddpik1/ddpik2 cells functioned normally in the regulation of other membrane traffic. For instance, radiolabel pulse-chase experiments indicated that the transport rates along the secretory pathway and the sorting efficiency of the lysosomal enzyme alpha-mannosidase were normal in the mutant strain. Furthermore, the contractile vacuole network of membranes (probably connected to the endosomal pathway by membrane traffic) was functionally and morphologically normal in mutant cells. Light microscopy revealed that delta ddpik1/ddpik2 cells appeared smaller and more irregularly shaped than wild-type cells; 1-3% of the mutant cells were also connected by a thin cytoplasmic bridge. Scanning EM indicated that the mutant cells contained numerous filopodia projecting laterally and vertically from the cell surface, and fluorescent microscopy indicated that these filopodia were enriched in F-actin which accumulated in a cortical pattern in control cells. Finally, delta ddpik1/ddpik2 cells responded and moved more rapidly towards cAMP. Together, these results suggest that Dictyostelium DdPIK1 and DdPIK2 gene products regulate multiple steps in the endosomal pathway, and function in the regulation of cell shape and movement perhaps through changes in actin organization.
Figures
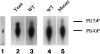
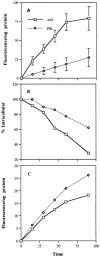
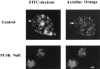
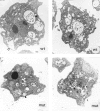
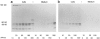
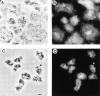
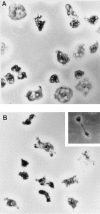
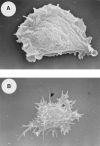
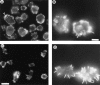
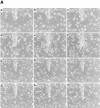
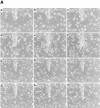
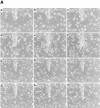
Similar articles
-
A phosphatidylinositol (PI) kinase gene family in Dictyostelium discoideum: biological roles of putative mammalian p110 and yeast Vps34p PI 3-kinase homologs during growth and development.Mol Cell Biol. 1995 Oct;15(10):5645-56. doi: 10.1128/MCB.15.10.5645. Mol Cell Biol. 1995. PMID: 7565716 Free PMC article.
-
A role for a Rab4-like GTPase in endocytosis and in regulation of contractile vacuole structure and function in Dictyostelium discoideum.Mol Biol Cell. 1996 Oct;7(10):1623-38. doi: 10.1091/mbc.7.10.1623. Mol Biol Cell. 1996. PMID: 8898366 Free PMC article.
-
p110-related PI 3-kinases regulate phagosome-phagosome fusion and phagosomal pH through a PKB/Akt dependent pathway in Dictyostelium.J Cell Sci. 2001 Apr;114(Pt 7):1283-95. doi: 10.1242/jcs.114.7.1283. J Cell Sci. 2001. PMID: 11256995
-
Dictyostelium discoideum: a genetic model system for the study of professional phagocytes. Profilin, phosphoinositides and the lmp gene family in Dictyostelium.Biochim Biophys Acta. 2001 Mar 15;1525(3):228-33. doi: 10.1016/s0304-4165(01)00108-8. Biochim Biophys Acta. 2001. PMID: 11257436 Review.
-
Molecular motors and membrane traffic in Dictyostelium.Biochim Biophys Acta. 2001 Mar 15;1525(3):234-44. doi: 10.1016/s0304-4165(01)00109-x. Biochim Biophys Acta. 2001. PMID: 11257437 Review.
Cited by
-
IQGAP-related protein IqgC suppresses Ras signaling during large-scale endocytosis.Proc Natl Acad Sci U S A. 2019 Jan 22;116(4):1289-1298. doi: 10.1073/pnas.1810268116. Epub 2019 Jan 8. Proc Natl Acad Sci U S A. 2019. PMID: 30622175 Free PMC article.
-
An SH2-domain-containing kinase negatively regulates the phosphatidylinositol-3 kinase pathway.Genes Dev. 2001 Mar 15;15(6):687-98. doi: 10.1101/gad.871001. Genes Dev. 2001. PMID: 11274054 Free PMC article.
-
The origins and evolution of macropinocytosis.Philos Trans R Soc Lond B Biol Sci. 2019 Feb 4;374(1765):20180158. doi: 10.1098/rstb.2018.0158. Philos Trans R Soc Lond B Biol Sci. 2019. PMID: 30967007 Free PMC article. Review.
-
Identification of a suppressor of the Dictyostelium profilin-minus phenotype as a CD36/LIMP-II homologue.J Cell Biol. 1999 Apr 5;145(1):167-81. doi: 10.1083/jcb.145.1.167. J Cell Biol. 1999. PMID: 10189376 Free PMC article.
-
Genetic control of morphogenesis in Dictyostelium.Dev Biol. 2015 Jun 15;402(2):146-61. doi: 10.1016/j.ydbio.2015.03.016. Epub 2015 Apr 11. Dev Biol. 2015. PMID: 25872182 Free PMC article. Review.
References
-
- Aubry L, Klein G, Martiel J, Satre M. Kinetics of endosomal pH evolution in Dictyostelium discoideumamoebae. J Cell Sci. 1993;105:861–866. - PubMed
-
- Bucci C, Parton R, Mather I, Stunnenberg H, Simons K, Hoflack B, Zerial M. The GTPase Rab 5 functions as a regulatory factor in the early endocytic pathway. Cell. 1992;70:715–728. - PubMed
Publication types
MeSH terms
Substances
Grants and funding
LinkOut - more resources
Full Text Sources
Molecular Biology Databases
Research Materials
Miscellaneous