Kinetics, Ca2+ dependence, and biophysical properties of integrin-mediated mechanical modulation of transmitter release from frog motor nerve terminals
- PMID: 8994045
- PMCID: PMC6573157
- DOI: 10.1523/JNEUROSCI.17-03-00904.1997
Kinetics, Ca2+ dependence, and biophysical properties of integrin-mediated mechanical modulation of transmitter release from frog motor nerve terminals
Abstract
Neurotransmitter release from frog motor nerve terminals is strongly modulated by change in muscle length. Over the physiological range, there is an approximately 10% increase in spontaneous and evoked release per 1% muscle stretch. Because many muscle fibers do not receive suprathreshold synaptic inputs at rest length, this stretch-induced enhancement of release constitutes a strong peripheral amplifier of the spinal stretch reflex. The stretch modulation of release is inhibited by peptides that block integrin binding of natural ligands. The modulation varies linearly with length, with a delay of no more than approximately 1-2 msec and is maintained constant at the new length. Moreover, the stretch modulation persists in a zero Ca2+ Ringer and, hence, is not dependent on Ca2+ influx through stretch activated channels. Eliminating transmembrane Ca2+ gradients and buffering intraterminal Ca2+ to approximately normal resting levels does not eliminate the modulation, suggesting that it is not the result of release of Ca2+ from internal stores. Finally, changes in temperature have no detectable effect on the kinetics of stretch-induced changes in endplate potential (EPP) amplitude or miniature EPP (mEPP) frequency. We conclude, therefore, that stretch does not act via second messenger pathways or a chemical modification of molecules involved in the release pathway. Instead, there is direct mechanical modulation of release. We postulate that tension on integrins in the presynaptic membrane is transduced mechanically into changes in the position or conformation of one or more molecules involved in neurotransmitter release, altering sensitivity to Ca2+ or the equilibrium for a critical reaction leading to vesicle fusion.
Figures
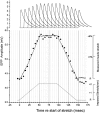
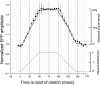
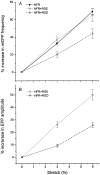
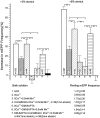
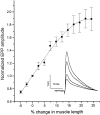
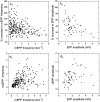
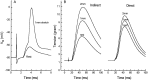
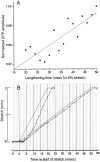
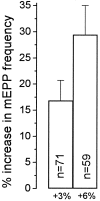
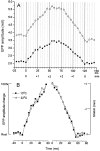
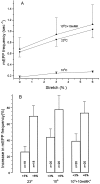
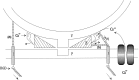
Similar articles
-
Hypertonic enhancement of transmitter release from frog motor nerve terminals: Ca2+ independence and role of integrins.J Physiol. 2001 Jan 15;530(Pt 2):243-52. doi: 10.1111/j.1469-7793.2001.0243l.x. J Physiol. 2001. PMID: 11208972 Free PMC article.
-
Integrins and modulation of transmitter release from motor nerve terminals by stretch.Science. 1995 Sep 15;269(5230):1578-80. doi: 10.1126/science.7667637. Science. 1995. PMID: 7667637
-
Regulation of transmitter release by muscle length in frog motor nerve terminals. Dynamics of the effect and the role of integrin-ECM interactions.Adv Second Messenger Phosphoprotein Res. 1994;29:383-98. Adv Second Messenger Phosphoprotein Res. 1994. PMID: 7848723 Review.
-
Dependence of spontaneous release at frog junctions on synaptic strength, external calcium and terminal length.J Physiol. 1989 Nov;418:397-410. doi: 10.1113/jphysiol.1989.sp017848. J Physiol. 1989. PMID: 2576068 Free PMC article.
-
The role of integrins in the modulation of neurotransmitter release from motor nerve terminals by stretch and hypertonicity.J Neurocytol. 2003 Jun-Sep;32(5-8):489-503. doi: 10.1023/B:NEUR.0000020606.58265.b5. J Neurocytol. 2003. PMID: 15034249 Review.
Cited by
-
Hypertonicity-induced transmitter release at Drosophila neuromuscular junctions is partly mediated by integrins and cAMP/protein kinase A.J Physiol. 2002 Jan 1;538(Pt 1):103-19. doi: 10.1113/jphysiol.2001.012901. J Physiol. 2002. PMID: 11773320 Free PMC article.
-
Cytoskeletal Mechanisms of Axonal Contractility.Biophys J. 2018 Aug 21;115(4):713-724. doi: 10.1016/j.bpj.2018.07.007. Epub 2018 Jul 12. Biophys J. 2018. PMID: 30054033 Free PMC article.
-
Activity-dependent regulation of synaptic AMPA receptor composition and abundance by beta3 integrins.Neuron. 2008 Jun 12;58(5):749-62. doi: 10.1016/j.neuron.2008.04.011. Neuron. 2008. PMID: 18549786 Free PMC article.
-
Mechanotransduction by intraganglionic laminar endings of vagal tension receptors in the guinea-pig oesophagus.J Physiol. 2003 Dec 1;553(Pt 2):575-87. doi: 10.1113/jphysiol.2003.051862. Epub 2003 Sep 18. J Physiol. 2003. PMID: 14500769 Free PMC article.
-
Temperature effect on carbachol-induced depression of spontaneous quantal transmitter release in frog neuromuscular junction.Neurochem Res. 2001 Sep;26(8-9):891-7. doi: 10.1023/a:1012372115058. Neurochem Res. 2001. PMID: 11699940
References
-
- Barrett EF, Magleby KL. Physiology of cholinergic transmission. In: Goldberg AM, Hanin I, editors. Biology of cholinergic function. Raven; New York: 1976. pp. 29–100.
-
- Chen BM, Grinnell AD. Integrins and modulation of transmitter release from motor nerve terminals by stretch. Science. 1995;269:1578–1580. - PubMed
-
- Cruz LJ, Gray WR, Olivera BM, Zeikus RD, Kerr L, Yoshikami D, Moczydlowski E. Conus geographus toxins that discriminate between neuronal and muscle sodium channels. J Biol Chem. 1985;260:9280–9288. - PubMed
MeSH terms
Substances
LinkOut - more resources
Full Text Sources
Miscellaneous