Axonal action-potential initiation and Na+ channel densities in the soma and axon initial segment of subicular pyramidal neurons
- PMID: 8824308
- PMCID: PMC6579266
- DOI: 10.1523/JNEUROSCI.16-21-06676.1996
Axonal action-potential initiation and Na+ channel densities in the soma and axon initial segment of subicular pyramidal neurons
Abstract
A long-standing hypothesis is that action potentials initiate first in the axon hillock/initial segment (AH-IS) region because of a locally high density of Na+ channels. We tested this idea in subicular pyramidal neurons by using patch-clamp recordings in hippocampal slices. Simultaneous recordings from the soma and IS confirmed that orthodromic action potentials initiated in the axon and then invaded the soma. However, blocking Na+ channels in the AH-IS with locally applied tetrodotoxin (TTX) did not raise the somatic threshold membrane potential for orthodromic spikes. TTX applied to the axon beyond the AH-IS (30-60 microm from the soma) raised the apparent somatic threshold by approximately 8 mV. We estimated the Na+ current density in the AH-IS and somatic membranes by using cell-attached patch-clamp recordings and found similar magnitudes (3-4 pA/microm2). Thus, the present results suggest that orthodromic action potentials initiate in the axon beyond the AH-IS and that the minimum threshold for spike initiation of the neuron is not determined by a high density of Na+ channels in the AH-IS region.
Figures
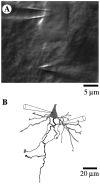
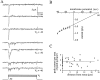
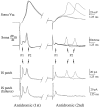
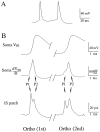
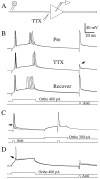
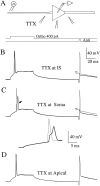
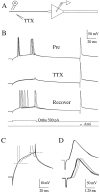
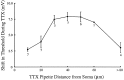
Similar articles
-
Involvement of persistent Na+ current in spike initiation in primary sensory neurons of the rat mesencephalic trigeminal nucleus.J Neurophysiol. 2007 Mar;97(3):2385-93. doi: 10.1152/jn.01191.2006. Epub 2007 Jan 17. J Neurophysiol. 2007. PMID: 17229822
-
Action potential initiation and propagation in rat neocortical pyramidal neurons.J Physiol. 1997 Dec 15;505 ( Pt 3)(Pt 3):617-32. doi: 10.1111/j.1469-7793.1997.617ba.x. J Physiol. 1997. PMID: 9457640 Free PMC article.
-
Autonomous initiation and propagation of action potentials in neurons of the subthalamic nucleus.J Physiol. 2008 Dec 1;586(23):5679-700. doi: 10.1113/jphysiol.2008.155861. Epub 2008 Oct 2. J Physiol. 2008. PMID: 18832425 Free PMC article.
-
Neuronal signaling in central nervous system.Sheng Li Xue Bao. 2011 Feb 25;63(1):1-8. Sheng Li Xue Bao. 2011. PMID: 21340428 Review.
-
Action potential initiation and propagation: upstream influences on neurotransmission.Neuroscience. 2009 Jan 12;158(1):211-22. doi: 10.1016/j.neuroscience.2008.03.021. Epub 2008 Mar 19. Neuroscience. 2009. PMID: 18472347 Free PMC article. Review.
Cited by
-
Dendritic ion channelopathy in acquired epilepsy.Epilepsia. 2012 Dec;53 Suppl 9(Suppl 9):32-40. doi: 10.1111/epi.12033. Epilepsia. 2012. PMID: 23216577 Free PMC article. Review.
-
Impaired axon initial segment structure and function in a model of ARHGEF9 developmental and epileptic encephalopathy.Proc Natl Acad Sci U S A. 2024 Oct 15;121(42):e2400709121. doi: 10.1073/pnas.2400709121. Epub 2024 Oct 7. Proc Natl Acad Sci U S A. 2024. PMID: 39374387
-
Specific and Plastic: Chandelier Cell-to-Axon Initial Segment Connections in Shaping Functional Cortical Network.Neurosci Bull. 2024 Nov;40(11):1774-1788. doi: 10.1007/s12264-024-01266-3. Epub 2024 Jul 30. Neurosci Bull. 2024. PMID: 39080101 Free PMC article. Review.
-
Electrogenic tuning of the axon initial segment.Neuroscientist. 2009 Dec;15(6):651-68. doi: 10.1177/1073858409341973. Neuroscientist. 2009. PMID: 20007821 Free PMC article. Review.
-
Na+ imaging reveals little difference in action potential-evoked Na+ influx between axon and soma.Nat Neurosci. 2010 Jul;13(7):852-60. doi: 10.1038/nn.2574. Epub 2010 Jun 13. Nat Neurosci. 2010. PMID: 20543843 Free PMC article.
References
-
- Adams PR. The platonic neuron gets the hots. Curr Biol. 1992;2:625–627. - PubMed
-
- Araki T, Otani T. Response of single motoneurons to direct stimulation in toad’s spinal cord. J Neurophysiol. 1955;18:472–485. - PubMed
-
- Benedeczky I, Molnár E, Somogyi P. The cisternal organelle as a Ca2+-storing compartment associated with GABAergic synapses in the axon initial segment of hippocampal pyramidal neurones. Exp Brain Res. 1994;101:216–230. - PubMed
-
- Black JA, Kocsis JD, Waxman SG. Ion channel organization of the myelinated fiber. Trends Neurosci. 1990;13:48–54. - PubMed
Publication types
MeSH terms
Substances
Grants and funding
LinkOut - more resources
Full Text Sources