The ATP-exporting channel Pannexin 1 promotes CD8+ T cell effector and memory responses
- PMID: 39045105
- PMCID: PMC11263643
- DOI: 10.1016/j.isci.2024.110290
The ATP-exporting channel Pannexin 1 promotes CD8+ T cell effector and memory responses
Abstract
Sensing of extracellular ATP (eATP) controls CD8+ T cell function. Their accumulation can occur through export by specialized molecules, such as the release channel Pannexin 1 (Panx1). Whether Panx1 controls CD8+ T cell immune responses in vivo, however, has not been previously addressed. Here, we report that T-cell-specific Panx1 is needed for CD8+ T cell responses to viral infections and cancer. We found that CD8-specific Panx1 promotes both effector and memory CD8+ T cell responses. Panx1 favors initial effector CD8+ T cell activation through extracellular ATP (eATP) export and subsequent P2RX4 activation, which helps promote full effector differentiation through extracellular lactate accumulation and its subsequent recycling. In contrast, Panx1 promotes memory CD8+ T cell survival primarily through ATP export and subsequent P2RX7 engagement, leading to improved mitochondrial metabolism. In summary, Panx1-mediated eATP export regulates effector and memory CD8+ T cells through distinct purinergic receptors and different metabolic and signaling pathways.
Keywords: Biochemistry; Biological sciences; Immune response; Immune system; Immunology; Natural sciences.
© 2024 The Author(s).
Conflict of interest statement
H.BdS. and N.L.T. are advisors for International Genomics Consortium. The remaining authors have no financial disclosures. The authors declare no competing interests.
Figures
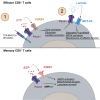
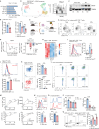
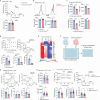
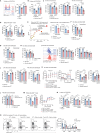
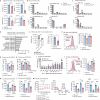
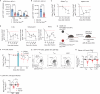
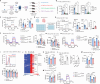
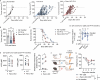
Update of
-
The ATP-exporting channel Pannexin-1 promotes CD8+ T cell effector and memory responses.bioRxiv [Preprint]. 2024 May 2:2023.04.19.537580. doi: 10.1101/2023.04.19.537580. bioRxiv. 2024. Update in: iScience. 2024 Jun 17;27(7):110290. doi: 10.1016/j.isci.2024.110290 PMID: 37131831 Free PMC article. Updated. Preprint.
Similar articles
-
The ATP-exporting channel Pannexin-1 promotes CD8+ T cell effector and memory responses.bioRxiv [Preprint]. 2024 May 2:2023.04.19.537580. doi: 10.1101/2023.04.19.537580. bioRxiv. 2024. Update in: iScience. 2024 Jun 17;27(7):110290. doi: 10.1016/j.isci.2024.110290 PMID: 37131831 Free PMC article. Updated. Preprint.
-
Self-Regulation of Memory CD8 T Cell Metabolism through Extracellular ATP Signaling.Immunometabolism. 2019;1(1):e190009. doi: 10.20900/immunometab20190009. Epub 2019 Jul 23. Immunometabolism. 2019. PMID: 31428464 Free PMC article.
-
The purinergic receptor P2RX7 directs metabolic fitness of long-lived memory CD8+ T cells.Nature. 2018 Jul;559(7713):264-268. doi: 10.1038/s41586-018-0282-0. Epub 2018 Jul 4. Nature. 2018. PMID: 29973721 Free PMC article.
-
Tissue- and temporal-specific roles of extracellular ATP on T cell metabolism and function.Immunometabolism (Cobham). 2023 May 1;5(2):e00025. doi: 10.1097/IN9.0000000000000025. eCollection 2023 Apr. Immunometabolism (Cobham). 2023. PMID: 37143525 Free PMC article. Review.
-
Pannexin: from discovery to bedside in 11±4 years?Brain Res. 2012 Dec 3;1487:150-9. doi: 10.1016/j.brainres.2012.04.058. Epub 2012 Jul 4. Brain Res. 2012. PMID: 22771709 Free PMC article. Review.
Cited by
-
Channel plan: control of adaptive immune responses by pannexins.Trends Immunol. 2024 Nov;45(11):892-902. doi: 10.1016/j.it.2024.09.009. Epub 2024 Oct 10. Trends Immunol. 2024. PMID: 39393945 Review.
-
CD39 Is Expressed on Functional Effector and Tissue-resident Memory CD8+ T Cells.J Immunol. 2024 Sep 1;213(5):588-599. doi: 10.4049/jimmunol.2400151. J Immunol. 2024. PMID: 38975728
References
-
- Milner J.J., Nguyen H., Omilusik K., Reina-Campos M., Tsai M., Toma C., Delpoux A., Boland B.S., Hedrick S.M., Chang J.T., Goldrath A.W. Delineation of a molecularly distinct terminally differentiated memory CD8 T cell population. Proc. Natl. Acad. Sci. USA. 2020;117:25667–25678. doi: 10.1073/pnas.2008571117. - DOI - PMC - PubMed
Grants and funding
LinkOut - more resources
Full Text Sources
Molecular Biology Databases
Research Materials
Miscellaneous