Stearoylation cycle regulates the cell surface distribution of the PCP protein Vangl2
- PMID: 38985771
- PMCID: PMC11260150
- DOI: 10.1073/pnas.2400569121
Stearoylation cycle regulates the cell surface distribution of the PCP protein Vangl2
Abstract
Defects in planar cell polarity (PCP) have been implicated in diverse human pathologies. Vangl2 is one of the core PCP components crucial for PCP signaling. Dysregulation of Vangl2 has been associated with severe neural tube defects and cancers. However, how Vangl2 protein is regulated at the posttranslational level has not been well understood. Using chemical reporters of fatty acylation and biochemical validation, here we present that Vangl2 subcellular localization is regulated by a reversible S-stearoylation cycle. The dynamic process is mainly regulated by acyltransferase ZDHHC9 and deacylase acyl-protein thioesterase 1 (APT1). The stearoylation-deficient mutant of Vangl2 shows decreased plasma membrane localization, resulting in disruption of PCP establishment during cell migration. Genetically or pharmacologically inhibiting ZDHHC9 phenocopies the effects of the stearoylation loss of Vangl2. In addition, loss of Vangl2 stearoylation enhances the activation of oncogenic Yes-associated protein 1 (YAP), serine-threonine kinase AKT, and extracellular signal-regulated protein kinase (ERK) signaling and promotes breast cancer cell growth and HRas G12V mutant (HRasV12)-induced oncogenic transformation. Our results reveal a regulation mechanism of Vangl2, and provide mechanistic insight into how fatty acid metabolism and protein fatty acylation regulate PCP signaling and tumorigenesis by core PCP protein lipidation.
Keywords: Vangl2; click chemistry; planar cell polarity; protein lipidation; stearoylation.
Conflict of interest statement
Competing interests statement:The authors declare no competing interest.
Figures
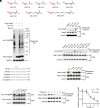
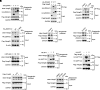
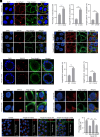
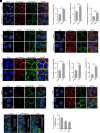
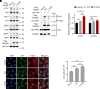
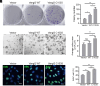
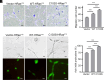
Similar articles
-
Functional analysis of germline VANGL2 variants using rescue assays of vangl2 knockout zebrafish.Hum Mol Genet. 2024 Jan 7;33(2):150-169. doi: 10.1093/hmg/ddad171. Hum Mol Genet. 2024. PMID: 37815931 Free PMC article.
-
Nuclear VANGL2 Inhibits Lactogenic Differentiation.Cells. 2024 Jan 25;13(3):222. doi: 10.3390/cells13030222. Cells. 2024. PMID: 38334614 Free PMC article.
-
Distinct functions of three Wnt proteins control mirror-symmetric organogenesis in the C. elegans gonad.Elife. 2024 Nov 1;13:e103035. doi: 10.7554/eLife.103035. Elife. 2024. PMID: 39485276 Free PMC article.
-
Depressing time: Waiting, melancholia, and the psychoanalytic practice of care.In: Kirtsoglou E, Simpson B, editors. The Time of Anthropology: Studies of Contemporary Chronopolitics. Abingdon: Routledge; 2020. Chapter 5. In: Kirtsoglou E, Simpson B, editors. The Time of Anthropology: Studies of Contemporary Chronopolitics. Abingdon: Routledge; 2020. Chapter 5. PMID: 36137063 Free Books & Documents. Review.
-
Wnt-signaling and planar cell polarity genes regulate axon guidance along the anteroposterior axis in C. elegans.Dev Neurobiol. 2014 Aug;74(8):781-96. doi: 10.1002/dneu.22146. Epub 2013 Dec 31. Dev Neurobiol. 2014. PMID: 24214205 Free PMC article. Review.
References
-
- Zallen J. A., Planar polarity and tissue morphogenesis. Cell 129, 1051–1063 (2007). - PubMed
-
- Luga V., et al. , Exosomes mediate stromal mobilization of autocrine Wnt-PCP signaling in breast cancer cell migration. Cell 151, 1542–1556 (2012). - PubMed
-
- Montcouquiol M., et al. , Identification of Vangl2 and Scrb1 as planar polarity genes in mammals. Nature 423, 173–177 (2003). - PubMed
MeSH terms
Substances
Grants and funding
LinkOut - more resources
Full Text Sources
Research Materials
Miscellaneous