Intron retention as a productive mechanism in human MAPT: RNA species generated by retention of intron 3
- PMID: 38181704
- PMCID: PMC10789595
- DOI: 10.1016/j.ebiom.2023.104953
Intron retention as a productive mechanism in human MAPT: RNA species generated by retention of intron 3
Abstract
Background: Tau is a microtubule-binding protein encoded by the MAPT gene. Tau is essential for several physiological functions and associated with pathological processes, including Alzheimer's disease (AD). Six tau isoforms are typically described in the central nervous system, but current research paints a more diverse landscape and a more nuanced balance between isoforms. Recent work has described tau isoforms generated by intron 11 and intron 12 retention. This work adds to that evidence, proving the existence of MAPT transcripts retaining intron 3. Our aim is to demonstrate the existence of mature MAPT RNA species that retain intron 3 in human brain samples and to study its correlation with Alzheimer's disease across different regions.
Methods: Initial evidence of intron-3-retaining MAPT species come from in silico analysis of RNA-seq databases. We further demonstrate the existence of these mature RNA species in a human neuroepithelioma cell line and human brain samples by quantitative PCR. We also use digital droplet PCR to demonstrate the existence of RNA species that retain either intron 3, intron 12 or both introns.
Findings: Intron-3-retaining species are even more prominently present that intron-12-retaining ones. We show the presence of MAPT transcripts that retain both introns 3 and 12. These intron-retaining species are diminished in brain samples of patients with Alzheimer's disease with respect to individuals without dementia. Conversely, relative abundance of intron-3- or intron-12-retaining MAPT species with respect to double-retaining species as well as their percentage of expression with respect to total MAPT are increased in patients with Alzheimer's disease, especially in hippocampal samples. Among these TIR-MAPT species, TIR3+12 double truncation allows better classification potential of Alzheimer's disease samples. Moreover, we find a significant increase in intron-3- or intron-12-retaining species and its relative abundance with respect to double-retaining MAPT species in cerebellum in contrast to frontal lateral cortex and hippocampus in individuals with no signs of dementia.
Interpretation: Intron retention constitutes a potential mechanism to generate Tau isoforms whose mature RNA expression levels correlate with Alzheimer's pathology showing its potential as a biomarker associated to the disease.
Funding: This research was funded by the Spanish Ministry of Science, Innovation and Universities: PGC2018-096177-B-I00 (J.A.); Spanish Ministry of Science and Innovation (MCIN): PID2020-113204GB-I00 (F.H.) and PID2021-123859OB-100 from MCIN/AEI/10.13039/501100011033/FEDER, UE (J.A.). It was also supported by CSIC through an intramural grant (201920E104) (J.A.) and the Centre for Networked Biomedical Research on Neurodegenerative Diseases (J.A.). The Centro de Biología Molecular Severo Ochoa (CBMSO) is a Severo Ochoa Center of Excellence (MICIN, award CEX2021-001154-S).
Keywords: Alternative splicing; Alzheimer's disease; Intron retention; Tau; Tau isoforms; Tauopathies.
Copyright © 2024 The Authors. Published by Elsevier B.V. All rights reserved.
Conflict of interest statement
Declaration of interests The authors declare no competing interests.
Figures
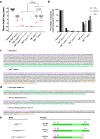
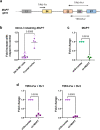
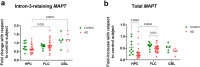
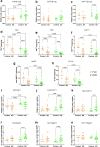
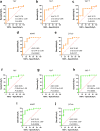
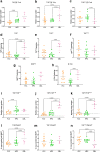
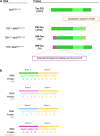
Similar articles
-
What's in a Gene? The Outstanding Diversity of MAPT.Cells. 2022 Mar 1;11(5):840. doi: 10.3390/cells11050840. Cells. 2022. PMID: 35269461 Free PMC article. Review.
-
A new non-aggregative splicing isoform of human Tau is decreased in Alzheimer's disease.Acta Neuropathol. 2021 Jul;142(1):159-177. doi: 10.1007/s00401-021-02317-z. Epub 2021 May 2. Acta Neuropathol. 2021. PMID: 33934221 Free PMC article.
-
Tau Isoforms: Gaining Insight into MAPT Alternative Splicing.Int J Mol Sci. 2022 Dec 6;23(23):15383. doi: 10.3390/ijms232315383. Int J Mol Sci. 2022. PMID: 36499709 Free PMC article. Review.
-
Truncated Tau caused by intron retention is enriched in Alzheimer's disease cortex and exhibits altered biochemical properties.Proc Natl Acad Sci U S A. 2022 Sep 13;119(37):e2204179119. doi: 10.1073/pnas.2204179119. Epub 2022 Sep 6. Proc Natl Acad Sci U S A. 2022. PMID: 36067305 Free PMC article.
-
Tau alternative splicing in familial and sporadic tauopathies.Biochem Soc Trans. 2012 Aug;40(4):677-80. doi: 10.1042/BST20120091. Biochem Soc Trans. 2012. PMID: 22817715 Review.
Cited by
-
Shared genetic links between hypothyroidism and psychiatric disorders: evidence from a comprehensive genetic analysis.Front Endocrinol (Lausanne). 2024 Jun 6;15:1370019. doi: 10.3389/fendo.2024.1370019. eCollection 2024. Front Endocrinol (Lausanne). 2024. PMID: 38904036 Free PMC article.
References
-
- Wang Y., Mandelkow E. Tau in physiology and pathology. Nat Rev Neurosci. 2016;17(1):22–35. - PubMed
-
- Avila J., Lucas J.J., Perez M., Hernandez F. Role of tau protein in both physiological and pathological conditions. Physiol Rev. 2004;84(2):361–384. - PubMed
-
- Buée L., Bussière T., Buée-Scherrer V., Delacourte A., Hof P.R. Tau protein isoforms, phosphorylation and role in neurodegenerative disorders. Brain Res Rev. 2000;33(1):95–130. - PubMed
-
- Andreadis A. Tau gene alternative splicing: expression patterns, regulation and modulation of function in normal brain and neurodegenerative diseases. Biochim Biophys Acta. 2005;1739(2-3):91–103. - PubMed
MeSH terms
Substances
LinkOut - more resources
Full Text Sources
Medical