Inducible MLL-AF9 Expression Drives an AML Program during Human Pluripotent Stem Cell-Derived Hematopoietic Differentiation
- PMID: 37190104
- PMCID: PMC10136707
- DOI: 10.3390/cells12081195
Inducible MLL-AF9 Expression Drives an AML Program during Human Pluripotent Stem Cell-Derived Hematopoietic Differentiation
Abstract
A t(9;11)(p22;q23) translocation produces the MLL-AF9 fusion protein, which is found in up to 25% of de novo AML cases in children. Despite major advances, obtaining a comprehensive understanding of context-dependent MLL-AF9-mediated gene programs during early hematopoiesis is challenging. Here, we generated a human inducible pluripotent stem cell (hiPSC) model with a doxycycline dose-dependent MLL-AF9 expression. We exploited MLL-AF9 expression as an oncogenic hit to uncover epigenetic and transcriptomic effects on iPSC-derived hematopoietic development and the transformation into (pre-)leukemic states. In doing so, we observed a disruption in early myelomonocytic development. Accordingly, we identified gene profiles that were consistent with primary MLL-AF9 AML and uncovered high-confidence MLL-AF9-associated core genes that are faithfully represented in primary MLL-AF9 AML, including known and presently unknown factors. Using single-cell RNA-sequencing, we identified an increase of CD34 expressing early hematopoietic progenitor-like cell states as well as granulocyte-monocyte progenitor-like cells upon MLL-AF9 activation. Our system allows for careful chemically controlled and stepwise in vitro hiPSC-derived differentiation under serum-free and feeder-free conditions. For a disease that currently lacks effective precision medicine, our system provides a novel entry-point into exploring potential novel targets for personalized therapeutic strategies.
Keywords: MLL-AF9; bioinformatics; differentiation; hematopoiesis; induced pluripotent stem cells (iPSCs); myeloid; oncogene.
Conflict of interest statement
The authors declare no conflict of interest.
Figures
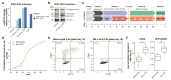
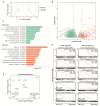
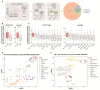
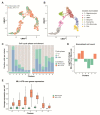
Similar articles
-
ZNF521 Enhances MLL-AF9-Dependent Hematopoietic Stem Cell Transformation in Acute Myeloid Leukemias by Altering the Gene Expression Landscape.Int J Mol Sci. 2021 Oct 6;22(19):10814. doi: 10.3390/ijms221910814. Int J Mol Sci. 2021. PMID: 34639154 Free PMC article.
-
Down-regulation of MLL-AF9, MLL and MYC expression is not obligatory for monocyte-macrophage maturation in AML-M5 cell lines carrying t(9;11)(p22;q23).Oncol Rep. 2006 Jan;15(1):207-11. Oncol Rep. 2006. PMID: 16328057
-
MLL-AF9 Expression in Hematopoietic Stem Cells Drives a Highly Invasive AML Expressing EMT-Related Genes Linked to Poor Outcome.Cancer Cell. 2016 Jul 11;30(1):43-58. doi: 10.1016/j.ccell.2016.05.011. Epub 2016 Jun 23. Cancer Cell. 2016. PMID: 27344946
-
Learning from mouse models of MLL fusion gene-driven acute leukemia.Biochim Biophys Acta Gene Regul Mech. 2020 Aug;1863(8):194550. doi: 10.1016/j.bbagrm.2020.194550. Epub 2020 Apr 19. Biochim Biophys Acta Gene Regul Mech. 2020. PMID: 32320749 Review.
-
The YEATS domain epigenetic reader proteins ENL and AF9 and their therapeutic value in leukemia.Exp Hematol. 2023 Aug;124:15-21. doi: 10.1016/j.exphem.2023.06.001. Epub 2023 Jun 7. Exp Hematol. 2023. PMID: 37295550 Free PMC article. Review.
Cited by
-
Understanding blood development and leukemia using sequencing-based technologies and human cell systems.Front Mol Biosci. 2023 Oct 10;10:1266697. doi: 10.3389/fmolb.2023.1266697. eCollection 2023. Front Mol Biosci. 2023. PMID: 37886034 Free PMC article. Review.
-
The Role of Epithelial-to-Mesenchymal Transition Transcription Factors (EMT-TFs) in Acute Myeloid Leukemia Progression.Biomedicines. 2024 Aug 21;12(8):1915. doi: 10.3390/biomedicines12081915. Biomedicines. 2024. PMID: 39200378 Free PMC article. Review.
References
Publication types
MeSH terms
Substances
Grants and funding
LinkOut - more resources
Full Text Sources
Medical