Stress-related biomolecular condensates in plants
- PMID: 37162152
- PMCID: PMC10473214
- DOI: 10.1093/plcell/koad127
Stress-related biomolecular condensates in plants
Abstract
Biomolecular condensates are membraneless organelle-like structures that can concentrate molecules and often form through liquid-liquid phase separation. Biomolecular condensate assembly is tightly regulated by developmental and environmental cues. Although research on biomolecular condensates has intensified in the past 10 years, our current understanding of the molecular mechanisms and components underlying their formation remains in its infancy, especially in plants. However, recent studies have shown that the formation of biomolecular condensates may be central to plant acclimation to stress conditions. Here, we describe the mechanism, regulation, and properties of stress-related condensates in plants, focusing on stress granules and processing bodies, 2 of the most well-characterized biomolecular condensates. In this regard, we showcase the proteomes of stress granules and processing bodies in an attempt to suggest methods for elucidating the composition and function of biomolecular condensates. Finally, we discuss how biomolecular condensates modulate stress responses and how they might be used as targets for biotechnological efforts to improve stress tolerance.
© The Author(s) 2023. Published by Oxford University Press on behalf of American Society of Plant Biologists.
Conflict of interest statement
Conflict of interest: The authors declare no conflict of interest.
Figures
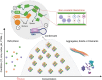
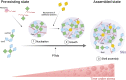
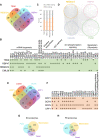
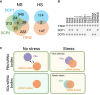
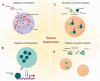
Similar articles
-
Biomolecular condensates in plant RNA silencing: insights into formation, function, and stress responses.Plant Cell. 2024 Jan 30;36(2):227-245. doi: 10.1093/plcell/koad254. Plant Cell. 2024. PMID: 37772963 Free PMC article. Review.
-
Using quantitative reconstitution to investigate multicomponent condensates.RNA. 2022 Jan;28(1):27-35. doi: 10.1261/rna.079008.121. Epub 2021 Nov 12. RNA. 2022. PMID: 34772789 Free PMC article. Review.
-
Targeting of biomolecular condensates to the autophagy pathway.Trends Cell Biol. 2023 Jun;33(6):505-516. doi: 10.1016/j.tcb.2022.08.006. Epub 2022 Sep 20. Trends Cell Biol. 2023. PMID: 36150962 Review.
-
Sodium ion influx regulates liquidity of biomolecular condensates in hyperosmotic stress response.Cell Rep. 2023 Apr 25;42(4):112315. doi: 10.1016/j.celrep.2023.112315. Epub 2023 Apr 4. Cell Rep. 2023. PMID: 37019112
-
Cajal bodies: Evolutionarily conserved nuclear biomolecular condensates with properties unique to plants.Plant Cell. 2023 Sep 1;35(9):3214-3235. doi: 10.1093/plcell/koad140. Plant Cell. 2023. PMID: 37202374 Free PMC article. Review.
Cited by
-
Liquid-liquid phase separation in plants: Advances and perspectives from model species to crops.Plant Commun. 2024 Jan 8;5(1):100663. doi: 10.1016/j.xplc.2023.100663. Epub 2023 Jul 26. Plant Commun. 2024. PMID: 37496271 Free PMC article. Review.
-
Overexpression of stress granule protein TZF1 enhances salt stress tolerance by targeting ACA11 mRNA for degradation in Arabidopsis.Front Plant Sci. 2024 May 8;15:1375478. doi: 10.3389/fpls.2024.1375478. eCollection 2024. Front Plant Sci. 2024. PMID: 38799098 Free PMC article.
-
Modulation of stress granule dynamics by phosphorylation and ubiquitination in plants.iScience. 2024 Oct 23;27(11):111162. doi: 10.1016/j.isci.2024.111162. eCollection 2024 Nov 15. iScience. 2024. PMID: 39569378 Free PMC article.
-
Silencing translation with phenolic acids.Nat Plants. 2023 Sep;9(9):1381-1382. doi: 10.1038/s41477-023-01497-8. Nat Plants. 2023. PMID: 37640932 No abstract available.
-
Liquid-liquid phase separation as a major mechanism of plant abiotic stress sensing and responses.Stress Biol. 2023 Dec 11;3(1):56. doi: 10.1007/s44154-023-00141-x. Stress Biol. 2023. PMID: 38078942 Free PMC article. Review.
References
Publication types
MeSH terms
Substances
LinkOut - more resources
Full Text Sources
Miscellaneous