The Nucleocapsid Proteins of SARS-CoV-2 and Its Close Relative Bat Coronavirus RaTG13 Are Capable of Inhibiting PKR- and RNase L-Mediated Antiviral Pathways
- PMID: 37154717
- PMCID: PMC10269842
- DOI: 10.1128/spectrum.00994-23
The Nucleocapsid Proteins of SARS-CoV-2 and Its Close Relative Bat Coronavirus RaTG13 Are Capable of Inhibiting PKR- and RNase L-Mediated Antiviral Pathways
Abstract
Coronaviruses (CoVs), including severe acute respiratory syndrome CoV (SARS-CoV), Middle East respiratory syndrome CoV (MERS-CoV), and SARS-CoV-2, produce double-stranded RNA (dsRNA) that activates antiviral pathways such as PKR and OAS/RNase L. To successfully replicate in hosts, viruses must evade such antiviral pathways. Currently, the mechanism of how SARS-CoV-2 antagonizes dsRNA-activated antiviral pathways is unknown. In this study, we demonstrate that the SARS-CoV-2 nucleocapsid (N) protein, the most abundant viral structural protein, is capable of binding to dsRNA and phosphorylated PKR, inhibiting both the PKR and OAS/RNase L pathways. The N protein of the bat coronavirus (bat-CoV) RaTG13, the closest relative of SARS-CoV-2, has a similar ability to inhibit the human PKR and RNase L antiviral pathways. Via mutagenic analysis, we found that the C-terminal domain (CTD) of the N protein is sufficient for binding dsRNA and inhibiting RNase L activity. Interestingly, while the CTD is also sufficient for binding phosphorylated PKR, the inhibition of PKR antiviral activity requires not only the CTD but also the central linker region (LKR). Thus, our findings demonstrate that the SARS-CoV-2 N protein is capable of antagonizing the two critical antiviral pathways activated by viral dsRNA and that its inhibition of PKR activities requires more than dsRNA binding mediated by the CTD. IMPORTANCE The high transmissibility of SARS-CoV-2 is an important viral factor defining the coronavirus disease 2019 (COVID-19) pandemic. To transmit efficiently, SARS-CoV-2 must be capable of disarming the innate immune response of its host efficiently. Here, we describe that the nucleocapsid protein of SARS-CoV-2 is capable of inhibiting two critical innate antiviral pathways, PKR and OAS/RNase L. Moreover, the counterpart of the closest animal coronavirus relative of SARS-CoV-2, bat-CoV RaTG13, can also inhibit human PKR and OAS/RNase L antiviral activities. Thus, the importance of our discovery for understanding the COVID-19 pandemic is 2-fold. First, the ability of SARS-CoV-2 N to inhibit innate antiviral activity is likely a factor contributing to the transmissibility and pathogenicity of the virus. Second, the bat relative of SARS-CoV-2 has the capacity to inhibit human innate immunity, which thus likely contributed to the establishment of infection in humans. The findings described in this study are valuable for developing novel antivirals and vaccines.
Keywords: G3BP1; PKR; RNase L; RaTG13; SARS-CoV-2 nucleocapsid; double-stranded RNA virus; vaccinia virus E3.
Conflict of interest statement
The authors declare no conflict of interest.
Figures
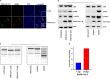
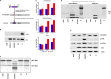
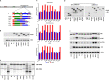
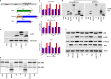
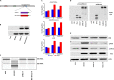
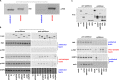
Similar articles
-
SARS-CoV-2 induces double-stranded RNA-mediated innate immune responses in respiratory epithelial-derived cells and cardiomyocytes.Proc Natl Acad Sci U S A. 2021 Apr 20;118(16):e2022643118. doi: 10.1073/pnas.2022643118. Proc Natl Acad Sci U S A. 2021. PMID: 33811184 Free PMC article.
-
SARS-CoV-2 induces double-stranded RNA-mediated innate immune responses in respiratory epithelial derived cells and cardiomyocytes.bioRxiv [Preprint]. 2020 Nov 2:2020.09.24.312553. doi: 10.1101/2020.09.24.312553. bioRxiv. 2020. Update in: Proc Natl Acad Sci U S A. 2021 Apr 20;118(16):e2022643118. doi: 10.1073/pnas.2022643118 PMID: 32995797 Free PMC article. Updated. Preprint.
-
Antagonism of dsRNA-Induced Innate Immune Pathways by NS4a and NS4b Accessory Proteins during MERS Coronavirus Infection.mBio. 2019 Mar 26;10(2):e00319-19. doi: 10.1128/mBio.00319-19. mBio. 2019. PMID: 30914508 Free PMC article.
-
Properties of Coronavirus and SARS-CoV-2.Malays J Pathol. 2020 Apr;42(1):3-11. Malays J Pathol. 2020. PMID: 32342926 Review.
-
Regulation of ribonucleoprotein condensates by RNase L during viral infection.Wiley Interdiscip Rev RNA. 2023 Jul-Aug;14(4):e1770. doi: 10.1002/wrna.1770. Epub 2022 Dec 7. Wiley Interdiscip Rev RNA. 2023. PMID: 36479619 Free PMC article. Review.
Cited by
-
Variant mutation in SARS-CoV-2 nucleocapsid enhances viral infection via altered genomic encapsidation.bioRxiv [Preprint]. 2024 Mar 11:2024.03.08.584120. doi: 10.1101/2024.03.08.584120. bioRxiv. 2024. PMID: 38559000 Free PMC article. Preprint.
-
Subsequent Waves of Convergent Evolution in SARS-CoV-2 Genes and Proteins.Vaccines (Basel). 2024 Aug 5;12(8):887. doi: 10.3390/vaccines12080887. Vaccines (Basel). 2024. PMID: 39204013 Free PMC article. Review.
-
Porcine deltacoronavirus nucleocapsid protein antagonizes JAK-STAT signaling pathway by targeting STAT1 through KPNA2 degradation.J Virol. 2024 Jul 23;98(7):e0033424. doi: 10.1128/jvi.00334-24. Epub 2024 Jun 3. J Virol. 2024. PMID: 38829137 Free PMC article.
References
-
- Wu F, Zhao S, Yu B, Chen Y-M, Wang W, Song Z-G, Hu Y, Tao Z-W, Tian J-H, Pei Y-Y, Yuan M-L, Zhang Y-L, Dai F-H, Liu Y, Wang Q-M, Zheng J-J, Xu L, Holmes EC, Zhang Y-Z. 2020. A new coronavirus associated with human respiratory disease in China. Nature 579:265–269. doi:10.1038/s41586-020-2008-3. - DOI - PMC - PubMed
-
- Holmes EC, Goldstein SA, Rasmussen AL, Robertson DL, Crits-Christoph A, Wertheim JO, Anthony SJ, Barclay WS, Boni MF, Doherty PC, Farrar J, Geoghegan JL, Jiang X, Leibowitz JL, Neil SJD, Skern T, Weiss SR, Worobey M, Andersen KG, Garry RF, Rambaut A. 2021. The origins of SARS-CoV-2: a critical review. Cell 184:4848–4856. doi:10.1016/j.cell.2021.08.017. - DOI - PMC - PubMed
Publication types
MeSH terms
Substances
Grants and funding
LinkOut - more resources
Full Text Sources
Medical
Miscellaneous