The SARS-CoV-2 accessory protein Orf3a is not an ion channel, but does interact with trafficking proteins
- PMID: 36695574
- PMCID: PMC9910834
- DOI: 10.7554/eLife.84477
The SARS-CoV-2 accessory protein Orf3a is not an ion channel, but does interact with trafficking proteins
Abstract
The severe acute respiratory syndrome associated coronavirus 2 (SARS-CoV-2) and SARS-CoV-1 accessory protein Orf3a colocalizes with markers of the plasma membrane, endocytic pathway, and Golgi apparatus. Some reports have led to annotation of both Orf3a proteins as viroporins. Here, we show that neither SARS-CoV-2 nor SARS-CoV-1 Orf3a form functional ion conducting pores and that the conductances measured are common contaminants in overexpression and with high levels of protein in reconstitution studies. Cryo-EM structures of both SARS-CoV-2 and SARS-CoV-1 Orf3a display a narrow constriction and the presence of a positively charged aqueous vestibule, which would not favor cation permeation. We observe enrichment of the late endosomal marker Rab7 upon SARS-CoV-2 Orf3a overexpression, and co-immunoprecipitation with VPS39. Interestingly, SARS-CoV-1 Orf3a does not cause the same cellular phenotype as SARS-CoV-2 Orf3a and does not interact with VPS39. To explain this difference, we find that a divergent, unstructured loop of SARS-CoV-2 Orf3a facilitates its binding with VPS39, a HOPS complex tethering protein involved in late endosome and autophagosome fusion with lysosomes. We suggest that the added loop enhances SARS-CoV-2 Orf3a's ability to co-opt host cellular trafficking mechanisms for viral exit or host immune evasion.
Keywords: HOPS complex; Orf3a; SARS-CoV; VPS39; cryo-EM; endocytic trafficking; ion channel; molecular biophysics; none; structural biology.
Conflict of interest statement
AM, PH, EM, DC, GL, BC, TD, SS, DM, RY, ZY, DR, SB, DC No competing interests declared
Figures
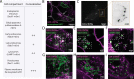
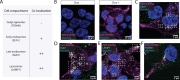
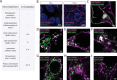
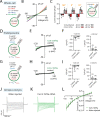
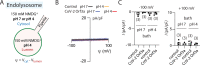
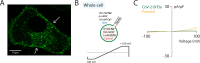
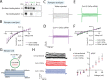
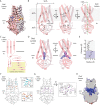
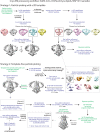
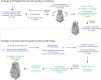
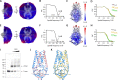
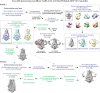
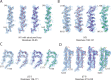

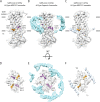
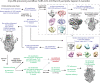
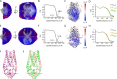
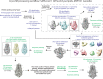
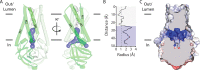
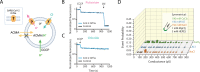
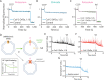
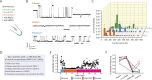

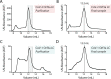
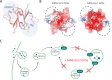
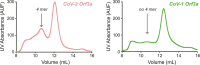
Update of
-
The SARS-CoV-2 accessory protein Orf3a is not an ion channel, but does interact with trafficking proteins.bioRxiv [Preprint]. 2022 Sep 3:2022.09.02.506428. doi: 10.1101/2022.09.02.506428. bioRxiv. 2022. Update in: Elife. 2023 Jan 25;12:e84477. doi: 10.7554/eLife.84477. PMID: 36263072 Free PMC article. Updated. Preprint.
Similar articles
-
The SARS-CoV-2 accessory protein Orf3a is not an ion channel, but does interact with trafficking proteins.bioRxiv [Preprint]. 2022 Sep 3:2022.09.02.506428. doi: 10.1101/2022.09.02.506428. bioRxiv. 2022. Update in: Elife. 2023 Jan 25;12:e84477. doi: 10.7554/eLife.84477. PMID: 36263072 Free PMC article. Updated. Preprint.
-
SARS-CoV-2 virulence factor ORF3a blocks lysosome function by modulating TBC1D5-dependent Rab7 GTPase cycle.Nat Commun. 2024 Mar 6;15(1):2053. doi: 10.1038/s41467-024-46417-2. Nat Commun. 2024. PMID: 38448435 Free PMC article.
-
ORF3a of the COVID-19 virus SARS-CoV-2 blocks HOPS complex-mediated assembly of the SNARE complex required for autolysosome formation.Dev Cell. 2021 Feb 22;56(4):427-442.e5. doi: 10.1016/j.devcel.2020.12.010. Epub 2020 Dec 16. Dev Cell. 2021. PMID: 33422265 Free PMC article.
-
Regulation of autophagy by SARS-CoV-2: The multifunctional contributions of ORF3a.J Med Virol. 2023 Jul;95(7):e28959. doi: 10.1002/jmv.28959. J Med Virol. 2023. PMID: 37485696 Review.
-
Structural biology of coronavirus ion channels.Acta Crystallogr D Struct Biol. 2021 Apr 1;77(Pt 4):391-402. doi: 10.1107/S2059798321001431. Epub 2021 Mar 3. Acta Crystallogr D Struct Biol. 2021. PMID: 33825700 Review.
Cited by
-
SARS-CoV-2 ORF 3a-mediated currents are inhibited by antiarrhythmic drugs.Europace. 2024 Oct 3;26(10):euae252. doi: 10.1093/europace/euae252. Europace. 2024. PMID: 39412366 Free PMC article.
-
Addressing SARS-CoV-2 viroporins with antiarrhythmic drugs.Europace. 2024 Oct 3;26(10):euae254. doi: 10.1093/europace/euae254. Europace. 2024. PMID: 39412365 Free PMC article. No abstract available.
-
SARS-CoV-2 ORF3a Protein Impairs Syncytiotrophoblast Maturation, Alters ZO-1 Localization, and Shifts Autophagic Pathways in Trophoblast Cells and 3D Organoids.bioRxiv [Preprint]. 2024 Sep 25:2024.09.25.614931. doi: 10.1101/2024.09.25.614931. bioRxiv. 2024. PMID: 39386577 Free PMC article. Preprint.
-
Adaptive truncation of the S gene in IBV during chicken embryo passaging plays a crucial role in its attenuation.PLoS Pathog. 2024 Jul 30;20(7):e1012415. doi: 10.1371/journal.ppat.1012415. eCollection 2024 Jul. PLoS Pathog. 2024. PMID: 39078847 Free PMC article.
-
Comprehensive proteomic analysis of HCoV-OC43 virions and virus-modulated extracellular vesicles.J Virol. 2024 Jul 23;98(7):e0085024. doi: 10.1128/jvi.00850-24. Epub 2024 Jul 2. J Virol. 2024. PMID: 38953378
References
Publication types
MeSH terms
Substances
Grants and funding
LinkOut - more resources
Full Text Sources
Medical
Miscellaneous