Molecular basis for antiviral activity of two pediatric neutralizing antibodies targeting SARS-CoV-2 Spike RBD
- PMID: 36514310
- PMCID: PMC9733284
- DOI: 10.1016/j.isci.2022.105783
Molecular basis for antiviral activity of two pediatric neutralizing antibodies targeting SARS-CoV-2 Spike RBD
Abstract
Neutralizing antibodies (NAbs) hold great promise for clinical interventions against SARS-CoV-2 variants of concern (VOCs). Understanding NAb epitope-dependent antiviral mechanisms is crucial for developing vaccines and therapeutics against VOCs. Here we characterized two potent NAbs, EH3 and EH8, isolated from an unvaccinated pediatric patient with exceptional plasma neutralization activity. EH3 and EH8 cross-neutralize the early VOCs and mediate strong Fc-dependent effector activity in vitro. Structural analyses of EH3 and EH8 in complex with the receptor-binding domain (RBD) revealed the molecular determinants of the epitope-driven protection and VOC evasion. While EH3 represents the prevalent IGHV3-53 NAb whose epitope substantially overlaps with the ACE2 binding site, EH8 recognizes a narrow epitope exposed in both RBD-up and RBD-down conformations. When tested in vivo, a single-dose prophylactic administration of EH3 fully protected stringent K18-hACE2 mice from lethal challenge with Delta VOC. Our study demonstrates that protective NAbs responses converge in pediatric and adult SARS-CoV-2 patients.
Keywords: Immunology; Virology.
© 2022 The Author(s).
Conflict of interest statement
A.F. has filed a provisional patent application on the following monoclonal antibodies: CV3-1 and CV3-25. A.F., J.P., V.L. M.A.S., V.-P.L., and E.H. have filed a provisional patent application on the following monoclonal antibodies: EH3 and EH8.
Figures
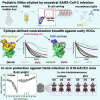
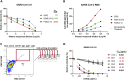
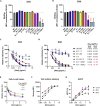
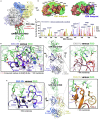
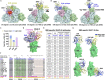
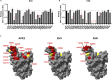
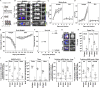
Similar articles
-
Effects of human anti-spike protein receptor binding domain antibodies on severe acute respiratory syndrome coronavirus neutralization escape and fitness.J Virol. 2014 Dec;88(23):13769-80. doi: 10.1128/JVI.02232-14. Epub 2014 Sep 17. J Virol. 2014. PMID: 25231316 Free PMC article.
-
Epitope Classification and RBD Binding Properties of Neutralizing Antibodies Against SARS-CoV-2 Variants of Concern.Front Immunol. 2021 Jun 4;12:691715. doi: 10.3389/fimmu.2021.691715. eCollection 2021. Front Immunol. 2021. PMID: 34149735 Free PMC article.
-
A Novel Nanobody Targeting Middle East Respiratory Syndrome Coronavirus (MERS-CoV) Receptor-Binding Domain Has Potent Cross-Neutralizing Activity and Protective Efficacy against MERS-CoV.J Virol. 2018 Aug 29;92(18):e00837-18. doi: 10.1128/JVI.00837-18. Print 2018 Sep 15. J Virol. 2018. PMID: 29950421 Free PMC article.
-
Structural Basis of a Human Neutralizing Antibody Specific to the SARS-CoV-2 Spike Protein Receptor-Binding Domain.Microbiol Spectr. 2021 Oct 31;9(2):e0135221. doi: 10.1128/Spectrum.01352-21. Epub 2021 Oct 13. Microbiol Spectr. 2021. PMID: 34643438 Free PMC article.
-
A Structural Landscape of Neutralizing Antibodies Against SARS-CoV-2 Receptor Binding Domain.Front Immunol. 2021 Apr 28;12:647934. doi: 10.3389/fimmu.2021.647934. eCollection 2021. Front Immunol. 2021. PMID: 33995366 Free PMC article. Review.
Cited by
-
Differentiating Cell Entry Potentials of SARS-CoV-2 Omicron Subvariants on Human Lung Epithelium Cells.Viruses. 2024 Mar 1;16(3):391. doi: 10.3390/v16030391. Viruses. 2024. PMID: 38543757 Free PMC article.
-
Bioluminescence imaging reveals enhanced SARS-CoV-2 clearance in mice with combinatorial regimens.iScience. 2024 Jan 30;27(3):109049. doi: 10.1016/j.isci.2024.109049. eCollection 2024 Mar 15. iScience. 2024. PMID: 38361624 Free PMC article.
-
The molecular basis of the neutralization breadth of the RBD-specific antibody CoV11.Front Immunol. 2023 Jun 2;14:1178355. doi: 10.3389/fimmu.2023.1178355. eCollection 2023. Front Immunol. 2023. PMID: 37334379 Free PMC article.
References
-
- Waterfield T., Watson C., Moore R., Ferris K., Tonry C., Watt A., McGinn C., Foster S., Evans J., Lyttle M.D., et al. Seroprevalence of SARS-CoV-2 antibodies in children: a prospective multicentre cohort study. Arch. Dis. Child. 2021;106:680–686. doi: 10.1136/archdischild-2020-320558. - DOI - PubMed
-
- Weisberg S.P., Connors T.J., Zhu Y., Baldwin M.R., Lin W.H., Wontakal S., Szabo P.A., Wells S.B., Dogra P., Gray J., et al. Distinct antibody responses to SARS-CoV-2 in children and adults across the COVID-19 clinical spectrum. Nat. Immunol. 2021;22:25–31. doi: 10.1038/s41590-020-00826-9. - DOI - PMC - PubMed
Grants and funding
LinkOut - more resources
Full Text Sources
Molecular Biology Databases
Miscellaneous