The Long-Term Pannexin 1 Ablation Produces Structural and Functional Modifications in Hippocampal Neurons
- PMID: 36429074
- PMCID: PMC9688914
- DOI: 10.3390/cells11223646
The Long-Term Pannexin 1 Ablation Produces Structural and Functional Modifications in Hippocampal Neurons
Abstract
Enhanced activity and overexpression of Pannexin 1 (Panx1) channels contribute to neuronal pathologies such as epilepsy and Alzheimer's disease (AD). The Panx1 channel ablation alters the hippocampus's glutamatergic neurotransmission, synaptic plasticity, and memory flexibility. Nevertheless, Panx1-knockout (Panx1-KO) mice still retain the ability to learn, suggesting that compensatory mechanisms stabilize their neuronal activity. Here, we show that the absence of Panx1 in the adult brain promotes a series of structural and functional modifications in the Panx1-KO hippocampal synapses, preserving spontaneous activity. Compared to the wild-type (WT) condition, the adult hippocampal neurons of Panx1-KO mice exhibit enhanced excitability, a more complex dendritic branching, enhanced spine maturation, and an increased proportion of multiple synaptic contacts. These modifications seem to rely on the actin-cytoskeleton dynamics as an increase in the actin polymerization and an imbalance between the Rac1 and the RhoA GTPase activities were observed in Panx1-KO brain tissues. Our findings highlight a novel interaction between Panx1 channels, actin, and Rho GTPases, which appear to be relevant for synapse stability.
Keywords: actin cytoskeleton; dendritic spines; neuronal morphology; pannexin 1.
Conflict of interest statement
The authors declare no conflict of interest.
Figures
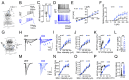
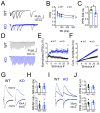
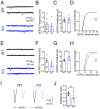
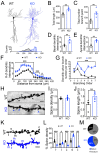
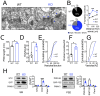
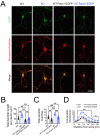
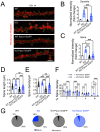
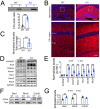
Similar articles
-
Pannexin-1 Modulates Inhibitory Transmission and Hippocampal Synaptic Plasticity.Biomolecules. 2023 May 25;13(6):887. doi: 10.3390/biom13060887. Biomolecules. 2023. PMID: 37371467 Free PMC article.
-
Pannexin 1 Regulates Network Ensembles and Dendritic Spine Development in Cortical Neurons.eNeuro. 2019 Jun 6;6(3):ENEURO.0503-18.2019. doi: 10.1523/ENEURO.0503-18.2019. Print 2019 May/Jun. eNeuro. 2019. PMID: 31118206 Free PMC article.
-
Astrocyte and Neuronal Panx1 Support Long-Term Reference Memory in Mice.ASN Neuro. 2023 Jan-Dec;15:17590914231184712. doi: 10.1177/17590914231184712. ASN Neuro. 2023. PMID: 37365910 Free PMC article.
-
Pannexin-1 channels in epilepsy.Neurosci Lett. 2019 Mar 16;695:71-75. doi: 10.1016/j.neulet.2017.09.004. Epub 2017 Sep 5. Neurosci Lett. 2019. PMID: 28886985 Review.
-
Pannexin-1 in the CNS: Emerging concepts in health and disease.J Neurochem. 2020 Sep;154(5):468-485. doi: 10.1111/jnc.15004. Epub 2020 Apr 6. J Neurochem. 2020. PMID: 32162337 Review.
Cited by
-
Pannexin-1 Modulates Inhibitory Transmission and Hippocampal Synaptic Plasticity.Biomolecules. 2023 May 25;13(6):887. doi: 10.3390/biom13060887. Biomolecules. 2023. PMID: 37371467 Free PMC article.
-
P2X7 receptors and pannexin1 hemichannels shape presynaptic transmission.Purinergic Signal. 2024 Jun;20(3):223-236. doi: 10.1007/s11302-023-09965-8. Epub 2023 Sep 15. Purinergic Signal. 2024. PMID: 37713157 Free PMC article. Review.
-
Overlap in synaptic neurological condition susceptibility pathways and the neural pannexin 1 interactome revealed by bioinformatics analyses.Channels (Austin). 2023 Dec;17(1):2253102. doi: 10.1080/19336950.2023.2253102. Epub 2023 Oct 8. Channels (Austin). 2023. PMID: 37807670 Free PMC article.
References
Publication types
MeSH terms
Substances
Grants and funding
LinkOut - more resources
Full Text Sources
Research Materials