ER as master regulator of membrane trafficking and organelle function
- PMID: 36108241
- PMCID: PMC9481738
- DOI: 10.1083/jcb.202205135
ER as master regulator of membrane trafficking and organelle function
Abstract
The endoplasmic reticulum (ER), which occupies a large portion of the cytoplasm, is the cell's main site for the biosynthesis of lipids and carbohydrate conjugates, and it is essential for folding, assembly, and biosynthetic transport of secreted proteins and integral membrane proteins. The discovery of abundant membrane contact sites (MCSs) between the ER and other membrane compartments has revealed that, in addition to its biosynthetic and secretory functions, the ER plays key roles in the regulation of organelle dynamics and functions. In this review, we will discuss how the ER regulates endosomes, lysosomes, autophagosomes, mitochondria, peroxisomes, and the Golgi apparatus via MCSs. Such regulation occurs via lipid and Ca2+ transfer and also via control of in trans dephosphorylation reactions and organelle motility, positioning, fusion, and fission. The diverse controls of other organelles via MCSs manifest the ER as master regulator of organelle biology.
© 2022 Wenzel et al.
Figures
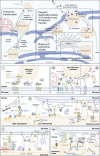
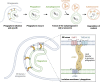
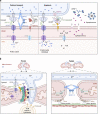
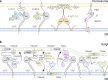
Similar articles
-
Organization and function of membrane contact sites.Biochim Biophys Acta. 2013 Nov;1833(11):2526-41. doi: 10.1016/j.bbamcr.2013.01.028. Epub 2013 Feb 1. Biochim Biophys Acta. 2013. PMID: 23380708 Review.
-
ER membranes exhibit phase behavior at sites of organelle contact.Proc Natl Acad Sci U S A. 2020 Mar 31;117(13):7225-7235. doi: 10.1073/pnas.1910854117. Epub 2020 Mar 16. Proc Natl Acad Sci U S A. 2020. PMID: 32179693 Free PMC article.
-
Here, there, and everywhere: The importance of ER membrane contact sites.Science. 2018 Aug 3;361(6401):eaan5835. doi: 10.1126/science.aan5835. Science. 2018. PMID: 30072511 Free PMC article. Review.
-
Structure and function of ER membrane contact sites with other organelles.Nat Rev Mol Cell Biol. 2016 Feb;17(2):69-82. doi: 10.1038/nrm.2015.8. Epub 2015 Dec 2. Nat Rev Mol Cell Biol. 2016. PMID: 26627931 Free PMC article. Review.
-
Lipid transfer and signaling at organelle contact sites: the tip of the iceberg.Curr Opin Cell Biol. 2011 Aug;23(4):458-63. doi: 10.1016/j.ceb.2011.04.006. Curr Opin Cell Biol. 2011. PMID: 21555211 Free PMC article. Review.
Cited by
-
Bibliometric analysis of endoplasmic reticulum stress in hepatocellular carcinoma: trends and future directions.Discov Oncol. 2024 Sep 27;15(1):481. doi: 10.1007/s12672-024-01377-3. Discov Oncol. 2024. PMID: 39331256 Free PMC article.
-
Closing the autophagosome is easy-PC.EMBO J. 2023 Jan 16;42(2):e113046. doi: 10.15252/embj.2022113046. Epub 2022 Dec 7. EMBO J. 2023. PMID: 36478568 Free PMC article.
-
LAMC2 mitigates ER stress by enhancing ER-mitochondria interaction via binding to MYH9 and MYH10.Cancer Gene Ther. 2024 Jan;31(1):43-57. doi: 10.1038/s41417-023-00680-5. Epub 2023 Oct 27. Cancer Gene Ther. 2024. PMID: 37891404 Free PMC article.
-
Novel functions of the ER-located Hsp40s DNAJB12 and DNAJB14 on proteins at the outer mitochondrial membrane under stress mediated by CCCP.Mol Cell Biochem. 2024 Oct;479(10):2637-2652. doi: 10.1007/s11010-023-04866-1. Epub 2023 Oct 18. Mol Cell Biochem. 2024. PMID: 37851175
-
Glycosphingolipids within membrane contact sites influence their function as signaling hubs in neurodegenerative diseases.FEBS Open Bio. 2023 Sep;13(9):1587-1600. doi: 10.1002/2211-5463.13605. Epub 2023 Apr 17. FEBS Open Bio. 2023. PMID: 37014126 Free PMC article. Review.
References
-
- Alanko, J., Hamidi H., and Ivaska J.. 2016. Signaling from endosomes. In Encyclopedia of Cell Biology. Bradshaw R.A., and Stahl P.D., editors. Academic Press, Waltham. 211–224
Publication types
MeSH terms
Substances
Grants and funding
LinkOut - more resources
Full Text Sources
Miscellaneous