Extracellular vesicles derived from patients with antibody-mediated rejection induce tubular senescence and endothelial to mesenchymal transition in renal cells
- PMID: 35583104
- PMCID: PMC9546277
- DOI: 10.1111/ajt.17097
Extracellular vesicles derived from patients with antibody-mediated rejection induce tubular senescence and endothelial to mesenchymal transition in renal cells
Abstract
Extracellular vesicles (EV) are emerging mediators in several diseases. However, their role in the pathophysiology of antibody-mediated allograft rejection (AMR) has been poorly investigated. Here, we investigated the role of EV isolated from AMR patients in inducing tubular senescence and endothelial to mesenchymal transition (EndMT) and analyzed their miRNA expression profile. By multiplex bead flow cytometry, we characterized the immunophenotype of plasma AMR-derived EV and found a prevalent platelet and endothelial cell origin. In vitro, AMR-derived EV induced tubular senescence by upregulating SA-β Gal and CDKN1A mRNA. Furthermore, AMR-derived EV induced EndMT. The occurrence of tubular senescence and EndMT was confirmed by analysis of renal biopsies from the same AMR patients. Moreover, AMR-derived EV induced C3 gene upregulation and CFH downregulation in tubular epithelial cells, with C4d deposition on endothelial cells. Interestingly, RNase-mediated digestion of EV cargo completely abrogated tubular senescence and EndMT. By microarray analysis, miR-604, miR-515-3p, miR-let-7d-5p, and miR-590-3p were significantly upregulated in EV from AMR group compared with transplant controls, whereas miR-24-3p and miR-29a-3p were downregulated. Therefore, EV-associated miRNA could act as active player in AMR pathogenesis, unraveling potential mechanisms of accelerated graft senescence, complement activation and early fibrosis that might lead to new therapeutic intervention.
Keywords: aging; antibody-mediated allograft rejection; cellular senescence; complement system; extracellular vesicles; miRNA.
© 2022 The Authors. American Journal of Transplantation published by Wiley Periodicals LLC on behalf of The American Society of Transplantation and the American Society of Transplant Surgeons.
Figures
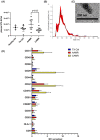
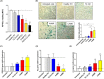
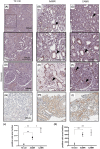
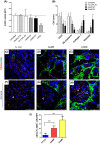
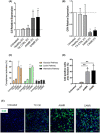
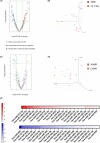
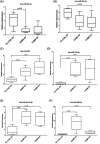
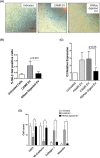
Comment in
-
Extracellular vesicles beyond biomarkers: Effectors of antibody-mediated rejection.Am J Transplant. 2022 Sep;22(9):2131-2132. doi: 10.1111/ajt.17133. Epub 2022 Jul 20. Am J Transplant. 2022. PMID: 35776663 No abstract available.
Similar articles
-
Endothelial Cell-Derived Extracellular Vesicles Target TLR4 via miRNA-326-3p to Regulate Skin Fibroblasts Senescence.J Immunol Res. 2022 May 18;2022:3371982. doi: 10.1155/2022/3371982. eCollection 2022. J Immunol Res. 2022. PMID: 35647205 Free PMC article.
-
IL-3R-alpha blockade inhibits tumor endothelial cell-derived extracellular vesicle (EV)-mediated vessel formation by targeting the β-catenin pathway.Oncogene. 2018 Mar;37(9):1175-1191. doi: 10.1038/s41388-017-0034-x. Epub 2017 Dec 14. Oncogene. 2018. PMID: 29238040 Free PMC article.
-
Extracellular Vesicles as Mediators of Cellular Crosstalk Between Immune System and Kidney Graft.Front Immunol. 2020 Feb 27;11:74. doi: 10.3389/fimmu.2020.00074. eCollection 2020. Front Immunol. 2020. PMID: 32180768 Free PMC article. Review.
-
Extracellular Vesicles from Fibroblasts Induce Epithelial-Cell Senescence in Pulmonary Fibrosis.Am J Respir Cell Mol Biol. 2020 Nov;63(5):623-636. doi: 10.1165/rcmb.2020-0002OC. Am J Respir Cell Mol Biol. 2020. PMID: 32730709
-
Effects of Replicative Senescence of Human Chorionic MSCs on their EV-miRNA Profile.Stem Cell Rev Rep. 2024 Nov;20(8):2318-2335. doi: 10.1007/s12015-024-10790-8. Epub 2024 Sep 21. Stem Cell Rev Rep. 2024. PMID: 39305404 Free PMC article.
Cited by
-
Emerging role of extracellular vesicles in kidney diseases.Front Pharmacol. 2022 Sep 12;13:985030. doi: 10.3389/fphar.2022.985030. eCollection 2022. Front Pharmacol. 2022. PMID: 36172178 Free PMC article. Review.
-
Blood-Brain Barrier Biomarkers before and after Kidney Transplantation.Int J Mol Sci. 2023 Apr 1;24(7):6628. doi: 10.3390/ijms24076628. Int J Mol Sci. 2023. PMID: 37047601 Free PMC article.
-
Exosomes on the development and progression of renal fibrosis.Cell Prolif. 2024 Nov;57(11):e13677. doi: 10.1111/cpr.13677. Epub 2024 Jun 19. Cell Prolif. 2024. PMID: 38898750 Free PMC article. Review.
-
New insights into maladaptive vascular responses to donor specific HLA antibodies in organ transplantation.Front Transplant. 2023 Apr 28;2:1146040. doi: 10.3389/frtra.2023.1146040. eCollection 2023. Front Transplant. 2023. PMID: 38993843 Free PMC article. Review.
-
Rictor/mTORC2 signalling contributes to renal vascular endothelial-to-mesenchymal transition and renal allograft interstitial fibrosis by regulating BNIP3-mediated mitophagy.Clin Transl Med. 2024 May;14(5):e1686. doi: 10.1002/ctm2.1686. Clin Transl Med. 2024. PMID: 38769658 Free PMC article.
References
-
- Théry C, Witwer KW, Aikawa E, et al. Minimal information for studies of extracellular vesicles 2018 (MISEV2018): a position statement of the International Society for Extracellular Vesicles and update of the MISEV2014 guidelines. J Extracell Vesicles. 2018;7(1):1535750. doi:10.1080/20013078.2018.1535750 - DOI - PMC - PubMed
Publication types
MeSH terms
Substances
LinkOut - more resources
Full Text Sources
Miscellaneous