Fabrication of SA/Gel/C scaffold with 3D bioprinting to generate micro-nano porosity structure for skin wound healing: a detailed animal in vivo study
- PMID: 35490207
- PMCID: PMC9056587
- DOI: 10.1186/s13619-022-00113-y
Fabrication of SA/Gel/C scaffold with 3D bioprinting to generate micro-nano porosity structure for skin wound healing: a detailed animal in vivo study
Abstract
Bioprinting has exhibited remarkable promises for the fabrication of functional skin substitutes. However, there are some significant challenges for the treatment of full-thickness skin defects in clinical practice. It is necessary to determine bioinks with suitable mechanical properties and desirable biocompatibilities. Additionally, the key for printing skin is to design the skin structure optimally, enabling the function of the skin. In this study, the full-thickness skin scaffolds were prepared with a gradient pore structure constructing the dense layer, epidermis, and dermis by different ratios of bioinks. We hypothesized that the dense layer protects the wound surface and maintains a moist environment on the wound surface. By developing a suitable hydrogel bioink formulation (sodium alginate/gelatin/collagen), to simulate the physiological structure of the skin via 3D printing, the proportion of hydrogels was optimized corresponding to each layer. These results reveal that the scaffold has interconnected macroscopic channels, and sodium alginate/gelatin/collagen scaffolds accelerated wound healing, reduced skin wound contraction, and re-epithelialization in vivo. It is expected to provide a rapid and economical production method of skin scaffolds for future clinical applications.
Keywords: 3D bioprinting; Hydrogel; Skin scaffold; Skin tissue engineering.
© 2022. The Author(s).
Conflict of interest statement
The authors declare that they have no known competing financial interests or personal relationships that could have appeared to influence the work reported in this paper.
Figures
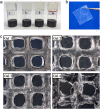
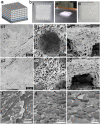
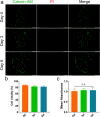
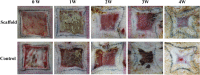
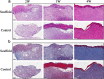
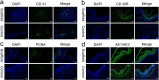
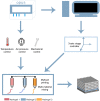
Similar articles
-
Three-dimensional bioprinting of a full-thickness functional skin model using acellular dermal matrix and gelatin methacrylamide bioink.Acta Biomater. 2021 Sep 1;131:248-261. doi: 10.1016/j.actbio.2021.07.012. Epub 2021 Jul 12. Acta Biomater. 2021. PMID: 34265473
-
Multifunctionalised skin substitute of hybrid gelatin-polyvinyl alcohol bioinks for chronic wound: injectable vs. 3D bioprinting.Drug Deliv Transl Res. 2024 Apr;14(4):1005-1027. doi: 10.1007/s13346-023-01447-z. Epub 2023 Nov 8. Drug Deliv Transl Res. 2024. PMID: 37938542
-
3D bioprinting of Salvianolic acid B-sodium alginate-gelatin skin scaffolds promotes diabetic wound repair via antioxidant, anti-inflammatory, and proangiogenic effects.Biomed Pharmacother. 2024 Feb;171:116168. doi: 10.1016/j.biopha.2024.116168. Epub 2024 Jan 16. Biomed Pharmacother. 2024. PMID: 38232662
-
Cellular Interaction of Human Skin Cells towards Natural Bioink via 3D-Bioprinting Technologies for Chronic Wound: A Comprehensive Review.Int J Mol Sci. 2022 Jan 1;23(1):476. doi: 10.3390/ijms23010476. Int J Mol Sci. 2022. PMID: 35008902 Free PMC article. Review.
-
Recent Advances in the Design of Three-Dimensional and Bioprinted Scaffolds for Full-Thickness Wound Healing.Tissue Eng Part B Rev. 2022 Feb;28(1):160-181. doi: 10.1089/ten.TEB.2020.0339. Epub 2021 Feb 22. Tissue Eng Part B Rev. 2022. PMID: 33446047 Review.
Cited by
-
A CS-based composite scaffold with excellent photothermal effect and its application in full-thickness skin wound healing.Regen Biomater. 2023 Apr 12;10:rbad028. doi: 10.1093/rb/rbad028. eCollection 2023. Regen Biomater. 2023. PMID: 37091498 Free PMC article.
-
Innovative Treatment Strategies to Accelerate Wound Healing: Trajectory and Recent Advancements.Cells. 2022 Aug 6;11(15):2439. doi: 10.3390/cells11152439. Cells. 2022. PMID: 35954282 Free PMC article. Review.
-
Alginate-Based Hydrogels and Scaffolds for Biomedical Applications.Mar Drugs. 2023 Mar 13;21(3):177. doi: 10.3390/md21030177. Mar Drugs. 2023. PMID: 36976226 Free PMC article. Review.
-
Application of Collagen-Based Hydrogel in Skin Wound Healing.Gels. 2023 Feb 27;9(3):185. doi: 10.3390/gels9030185. Gels. 2023. PMID: 36975634 Free PMC article. Review.
-
3D-bioprinted human lipoaspirate-derived cell-laden skin constructs for healing of full-thickness skin defects.Int J Bioprint. 2023 Mar 23;9(4):718. doi: 10.18063/ijb.718. eCollection 2023. Int J Bioprint. 2023. PMID: 37323499 Free PMC article.
References
-
- Admane P, Gupta AC, Jois P, Roy S, Chandrasekharan Lakshmanan C, Kalsi G, Bandyopadhyay B, Ghosh S. Direct 3D bioprinted full-thickness skin constructs recapitulate regulatory signaling pathways and physiology of human skin. Bioprinting. 2019;15:e00051. doi: 10.1016/j.bprint.2019.e00051. - DOI
-
- Albanna M, Binder KW, Murphy SV, Kim J, Qasem SA, Zhao W, Tan J, El-Amin IB, Dice DD, Marco J, Green J, Xu T, Skardal A, Holmes JH, Jackson JD, Atala A, Yoo JJ. In Situ Bioprinting of Autologous Skin Cells Accelerates Wound Healing of Extensive Excisional Full-Thickness Wounds. Sci Rep. 2019;9(1):1856. doi: 10.1038/s41598-018-38366-w. - DOI - PMC - PubMed
-
- Beheshtizadeh N, Lotfibakhshaiesh N, Pazhouhnia Z, Hoseinpour M, Nafari M. A review of 3D bio-printing for bone and skin tissue engineering: a commercial approach. J Mater Sci. 2019;55(9):3729–3749. doi: 10.1007/s10853-019-04259-0. - DOI
Grants and funding
- 2018YFA0703100/Key Technologies Research and Development Program
- BE2017664/Jiangsu Provincial Key Research and Development Program
- YG2017QN15/Shanghai Jiao Tong University Biomedical Engineering Cross Research Foundation
- 82072217/National Natural Science Foundation of China
- 81772135/National Natural Science Foundation of China
LinkOut - more resources
Full Text Sources