Heparan Sulfate, Mucopolysaccharidosis IIIB and Sulfur Metabolism Disorders
- PMID: 35453363
- PMCID: PMC9026333
- DOI: 10.3390/antiox11040678
Heparan Sulfate, Mucopolysaccharidosis IIIB and Sulfur Metabolism Disorders
Abstract
Mucopolysaccharidosis, type IIIB (MPS IIIB) is a rare disease caused by mutations in the N-alpha-acetylglucosaminidase (NAGLU) gene resulting in decreased or absent enzyme activity. On the cellular level, the disorder is characterized by the massive lysosomal storage of heparan sulfate (HS)-one species of glycosaminoglycans. HS is a sulfur-rich macromolecule, and its accumulation should affect the turnover of total sulfur in cells; according to the studies presented here, it, indeed, does. The lysosomal degradation of HS in cells produces monosaccharides and inorganic sulfate (SO42-). Sulfate is a product of L-cysteine metabolism, and any disruption of its levels affects the entire L-cysteine catabolism pathway, which was first reported in 2019. It is known that L-cysteine level is elevated in cells with the Naglu-/- gene mutation and in selected tissues of individuals with MPS IIIB. The level of glutathione and the Naglu-/- cells' antioxidant potential are significantly reduced, as well as the activity of 3-mercaptopyruvate sulfurtransferase (MPST, EC 2.8.1.2) and the level of sulfane sulfur-containing compounds. The direct reason is not yet known. This paper attempts to identify some of cause-and-effect correlations that may lead to this condition and identifies research directions that should be explored.
Keywords: 3-mercaptopyruvate sulfurtransferase; Sanfilippo B syndrome; cysteine; glycosaminoglycans; heparin; sulfane sulfur; sulfate; sulfurtransferases.
Conflict of interest statement
The authors declare no conflict of interest.
Figures
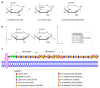
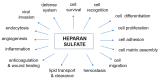
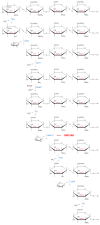
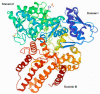
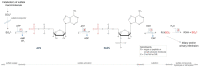
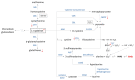
Similar articles
-
Effect of glycosaminoglycans accumulation on the non-oxidative sulfur metabolism in mouse model of Sanfilippo syndrome, type B.Acta Biochim Pol. 2019 Dec 5;66(4):567-576. doi: 10.18388/abp.2019_2880. Acta Biochim Pol. 2019. PMID: 31805227
-
Murine cellular model of mucopolysaccharidosis, type IIIB (MPS IIIB) - A preliminary study with particular emphasis on the non-oxidative l-cysteine metabolism.Biochimie. 2020 Jul;174:84-94. doi: 10.1016/j.biochi.2020.04.015. Epub 2020 Apr 23. Biochimie. 2020. PMID: 32335228
-
Differential Uptake of NAGLU-IGF2 and Unmodified NAGLU in Cellular Models of Sanfilippo Syndrome Type B.Mol Ther Methods Clin Dev. 2019 May 24;14:56-63. doi: 10.1016/j.omtm.2019.05.008. eCollection 2019 Sep 13. Mol Ther Methods Clin Dev. 2019. PMID: 31309128 Free PMC article.
-
Molecular genetics of mucopolysaccharidosis type IIIA and IIIB: Diagnostic, clinical, and biological implications.Hum Mutat. 2001 Oct;18(4):264-81. doi: 10.1002/humu.1189. Hum Mutat. 2001. PMID: 11668611 Review.
-
Glycosaminoglycans and mucopolysaccharidosis type III.Front Biosci (Landmark Ed). 2016 Jun 1;21(7):1393-409. doi: 10.2741/4463. Front Biosci (Landmark Ed). 2016. PMID: 27100513 Review.
Cited by
-
Dimethyl Sulfoxide (DMSO) as a Potential Source of Interference in Research Related to Sulfur Metabolism-A Preliminary Study.Antioxidants (Basel). 2024 May 9;13(5):582. doi: 10.3390/antiox13050582. Antioxidants (Basel). 2024. PMID: 38790687 Free PMC article.
-
Mucopolysaccharidosis type IIIB: a current review and exploration of the AAV therapy landscape.Neural Regen Res. 2024 Feb;19(2):355-359. doi: 10.4103/1673-5374.377606. Neural Regen Res. 2024. PMID: 37488890 Free PMC article. Review.
-
Sanfilippo Syndrome: Optimizing Care with a Multidisciplinary Approach.J Multidiscip Healthc. 2022 Sep 19;15:2097-2110. doi: 10.2147/JMDH.S362994. eCollection 2022. J Multidiscip Healthc. 2022. PMID: 36158637 Free PMC article. Review.
-
Evaluation of Intrafollicular Syndecan 1, Glypican 3, and Spermidine Levels in Women with Diminished Ovarian Reserve.Reprod Sci. 2023 Feb;30(2):569-575. doi: 10.1007/s43032-022-01085-9. Epub 2022 Sep 21. Reprod Sci. 2023. PMID: 36131221
-
Reactive Sulfur Species (RSS) in Physiological and Pathological Conditions and in Therapy.Antioxidants (Basel). 2022 Aug 15;11(8):1576. doi: 10.3390/antiox11081576. Antioxidants (Basel). 2022. PMID: 36009294 Free PMC article.
References
-
- Regulation (EC) No 141/2000 of the European Parliament and of the Council of 16 December 1999 on Orphan Medicinal Products. European Union; Maastricht, The Netherlands: 2000. pp. 1–5. The Official Journal of the European Union.
-
- Kan S.H., Aoyagai-Scharber M., Le S.Q., Vincelette J., Ohmi K., Bullens S., Wendt D.J., Christianson T.M., Tiger P.M.N., Brown J.R., et al. Delivery of an enzyme-IGFII fusion protein to the mouse brain is therapeutic for mucopolysaccharidosis type IIIB. Proc. Natl. Acad. Sci. USA. 2014;111:14870–14875. doi: 10.1073/pnas.1416660111. - DOI - PMC - PubMed
-
- Kan S.H., Troitskaya L.A., Sinow C.S., Haitz K., Todd A.K., Di Stefano A., Le S.Q., Dickson P.I., Tippin B.L. Insulin-like growth factor II peptide fusion enables uptake and lysosomal delivery of α-N-acetylglucosaminidase to mucopolysaccharidosis type IIIB fibroblasts. Biochem. J. 2014;458:281–289. doi: 10.1042/BJ20130845. - DOI - PMC - PubMed
Publication types
Grants and funding
LinkOut - more resources
Full Text Sources