Everolimus Alleviates Renal Allograft Interstitial Fibrosis by Inhibiting Epithelial-to-Mesenchymal Transition Not Only via Inducing Autophagy but Also via Stabilizing IκB-α
- PMID: 35140705
- PMCID: PMC8818677
- DOI: 10.3389/fimmu.2021.753412
Everolimus Alleviates Renal Allograft Interstitial Fibrosis by Inhibiting Epithelial-to-Mesenchymal Transition Not Only via Inducing Autophagy but Also via Stabilizing IκB-α
Abstract
Chronic allograft dysfunction (CAD) is the major cause of late graft loss in long-term renal transplantation. In our previous study, we found that epithelial-mesenchymal transition (EMT) is a significant event in the progression of renal allograft tubulointerstitial fibrosis, and impaired autophagic flux plays a critical role in renal allograft fibrosis. Everolimus (EVR) has been reported to be widely used to prevent the progression of organ fibrosis and graft rejection. However, the pharmacological mechanism of EVR in kidney transplantation remains to be determined. We used CAD rat model and the human kidney 2 (HK2) cell line treated with tumor necrosis factor-α (TNF-α) and EVR to examine the role of EVR on TNF-α-induced EMT and transplanted renal interstitial fibrosis. Here, we found that EVR could attenuate the progression of EMT and renal allograft interstitial fibrosis, and also activate autophagy in vivo. To explore the mechanism behind it, we detected the relationship among EVR, autophagy level, and TNF-α-induced EMT in HK2 cells. Our results showed that autophagy was upregulated upon mTOR pathway inhibition by EVR, which could significantly reduce expression of TNF-α-induced EMT. However, the inhibition of EVR on TNF-α-induced EMT was partly reversed following the addition of autophagy inhibitor chloroquine. In addition, we found that TNF-α activated EMT through protein kinase B (Akt) as well as nuclear factor kappa B (NF-κB) pathway according to the RNA sequencing, and EVR's effect on the EMT was only associated with IκB-α stabilization instead of the Akt pathway. Together, our findings suggest that EVR may retard impaired autophagic flux and block NF-κB pathway activation, and thereby prevent progression of TNF-α-induced EMT and renal allograft interstitial fibrosis.
Keywords: EMT; autophagy; chronic renal graft dysfunction; everolimus; renal allograft interstitial fibrosis.
Copyright © 2022 Gui, Suo, Tao, Wang, Zheng, Fei, Chen, Sun, Han, Ju, Zhang, Gu and Tan.
Conflict of interest statement
The authors declare that the research was conducted in the absence of any commercial or financial relationships that could be construed as a potential conflict of interest.
Figures
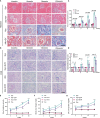
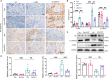
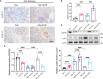
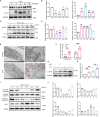
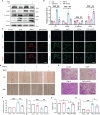
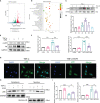
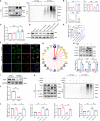
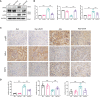
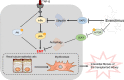
Similar articles
-
Bortezomib limits renal allograft interstitial fibrosis by inhibiting NF-κB/TNF-α/Akt/mTOR/P70S6K/Smurf2 pathway via IκBα protein stabilization.Clin Sci (Lond). 2021 Jan 15;135(1):53-69. doi: 10.1042/CS20201038. Clin Sci (Lond). 2021. PMID: 33289516
-
Bortezomib attenuates renal interstitial fibrosis in kidney transplantation via regulating the EMT induced by TNF-α-Smurf1-Akt-mTOR-P70S6K pathway.J Cell Mol Med. 2019 Aug;23(8):5390-5402. doi: 10.1111/jcmm.14420. Epub 2019 May 29. J Cell Mol Med. 2019. PMID: 31140729 Free PMC article.
-
Impaired ATG16L-Dependent Autophagy Promotes Renal Interstitial Fibrosis in Chronic Renal Graft Dysfunction Through Inducing EndMT by NF-κB Signal Pathway.Front Immunol. 2021 Apr 13;12:650424. doi: 10.3389/fimmu.2021.650424. eCollection 2021. Front Immunol. 2021. PMID: 33927720 Free PMC article.
-
Epithelial-to-mesenchymal transition and chronic allograft tubulointerstitial fibrosis.Transplant Rev (Orlando). 2008 Jan;22(1):1-5. doi: 10.1016/j.trre.2007.09.004. Transplant Rev (Orlando). 2008. PMID: 18631853 Free PMC article. Review.
-
Pathogenesis of tubulointerstitial fibrosis in chronic allograft dysfunction.Clin Transplant. 2009 Dec;23 Suppl 21:26-32. doi: 10.1111/j.1399-0012.2009.01106.x. Clin Transplant. 2009. PMID: 19930313 Review.
Cited by
-
Network pharmacology and experimental verification to decode the action of Qing Fei Hua Xian Decotion against pulmonary fibrosis.PLoS One. 2024 Jun 24;19(6):e0305903. doi: 10.1371/journal.pone.0305903. eCollection 2024. PLoS One. 2024. PMID: 38913698 Free PMC article.
-
PTB Regulates Keloid Fibroblast Migration and Proliferation Through Autophagy.Aesthetic Plast Surg. 2024 Oct 14. doi: 10.1007/s00266-024-04375-6. Online ahead of print. Aesthetic Plast Surg. 2024. PMID: 39402202
-
Rictor/mTORC2 signalling contributes to renal vascular endothelial-to-mesenchymal transition and renal allograft interstitial fibrosis by regulating BNIP3-mediated mitophagy.Clin Transl Med. 2024 May;14(5):e1686. doi: 10.1002/ctm2.1686. Clin Transl Med. 2024. PMID: 38769658 Free PMC article.
-
Tanshinone IIA is superior to paricalcitol in ameliorating tubulointerstitial fibrosis through regulation of VDR/Wnt/β-catenin pathway in rats with diabetic nephropathy.Naunyn Schmiedebergs Arch Pharmacol. 2024 Jun;397(6):3959-3977. doi: 10.1007/s00210-023-02853-3. Epub 2023 Nov 22. Naunyn Schmiedebergs Arch Pharmacol. 2024. PMID: 37991543 Free PMC article.
-
DNA methyltransferase 1 knockdown reverses PTEN and VDR by mediating demethylation of promoter and protects against renal injuries in hepatitis B virus-associated glomerulonephritis.Cell Biosci. 2022 Jun 28;12(1):98. doi: 10.1186/s13578-022-00835-1. Cell Biosci. 2022. PMID: 35765066 Free PMC article.
References
Publication types
MeSH terms
Substances
LinkOut - more resources
Full Text Sources
Miscellaneous