In situ synthesis of hydroxyapatite nanorods on graphene oxide nanosheets and their reinforcement in biopolymer scaffold
- PMID: 35024192
- PMCID: PMC8721358
- DOI: 10.1016/j.jare.2021.03.009
In situ synthesis of hydroxyapatite nanorods on graphene oxide nanosheets and their reinforcement in biopolymer scaffold
Abstract
Introduction: It is urgently needed to develop composite bone scaffold with excellent mechanical properties and bioactivity in bone tissue engineering. Combining graphene oxide (GO) and hydroxyapatite (HAP) for the reinforcement of biopolymer bone scaffold has emerged as a promising strategy. However, the dispersion of GO and HAP remains to be a big challenge.
Objectives: In this present work, the mechanical properties of GO and the bioactivity of and HAP were combined respectively via in situ synthesis for reinforcing biopolymer bone scaffold.
Methods: GO nanosheets were employed to in situ synthesize GO-HAP nanocomposite via hydrothermal reaction, in which their abundant oxygen-containing groups served as anchor sites for the chelation of Ca2+ and then Ca2+ absorbed HPO42- via electrovalent bonding to form homogeneously dispersed HAP nanorods. Thereby, the GO-HAP nanocomposite was blended with biopolymer poly-L-lactic acid (PLLA) for fabricating biopolymer scaffold by selective laser sintering (SLS).
Results: GO nanosheets were uniformly decorated with HAP nanorods, which were about 60 nm in length and 5 nm in diameter. The compressive strength and modulus of PLLA/12%GO-HAP were significantly increased by 53.71% and 98.80% compared to the pure PLLA scaffold, respectively, explained on the base of pull out, crack bridging, deflection and pinning mechanisms. Meanwhile, the mineralization experiments indicated the PLLA/GO-HAP scaffold displayed good bioactivity by inducing the formation of apatite layer. Besides, cell culturing experiments demonstrated the favorable cytocompatibility of scaffold by promoting cell adhesion and proliferation.
Conclusions: The present findings show the potential of PLLA/GO-HAP composite scaffold via in situ synthesis in bone tissue engineering.
Keywords: Bioactivity; GO; HAP; In situ synthesis; Mechanical properties.
© 2021 The Authors. Published by Elsevier B.V. on behalf of Cairo University.
Conflict of interest statement
The authors declare that they have no known competing financial interests or personal relationships that could have appeared to influence the work reported in this paper.
Figures
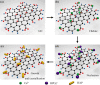
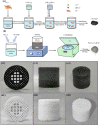
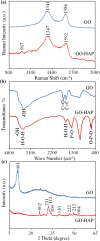
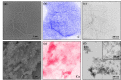
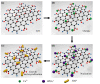
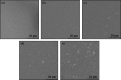
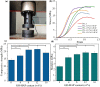
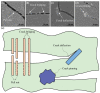
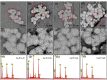
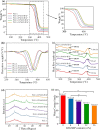
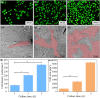
Similar articles
-
In Situ Generation of Hydroxyapatite on Biopolymer Particles for Fabrication of Bone Scaffolds Owning Bioactivity.ACS Appl Mater Interfaces. 2020 Oct 14;12(41):46743-46755. doi: 10.1021/acsami.0c13768. Epub 2020 Sep 30. ACS Appl Mater Interfaces. 2020. PMID: 32940994
-
Construction of a stereocomplex between poly(D-lactide) grafted hydroxyapatite and poly(L-lactide): toward a bioactive composite scaffold with enhanced interfacial bonding.J Mater Chem B. 2022 Jan 5;10(2):214-223. doi: 10.1039/d1tb02111g. J Mater Chem B. 2022. PMID: 34927656
-
Silicon dioxide nanoparticles decorated on graphene oxide nanosheets and their application in poly(l-lactic acid) scaffold.J Adv Res. 2023 Jun;48:175-190. doi: 10.1016/j.jare.2022.08.017. Epub 2022 Sep 7. J Adv Res. 2023. PMID: 36087925 Free PMC article.
-
Characterizations and interfacial reinforcement mechanisms of multicomponent biopolymer based scaffold.Mater Sci Eng C Mater Biol Appl. 2019 Jul;100:809-825. doi: 10.1016/j.msec.2019.03.030. Epub 2019 Mar 18. Mater Sci Eng C Mater Biol Appl. 2019. PMID: 30948118 Review.
-
[Advances in the Application of Hydroxyapatite Composite Materials in Bone Tissue Engineering].Sichuan Da Xue Xue Bao Yi Xue Ban. 2021 May;52(3):357-363. doi: 10.12182/20210560303. Sichuan Da Xue Xue Bao Yi Xue Ban. 2021. PMID: 34018351 Free PMC article. Review. Chinese.
Cited by
-
Preparation and characterization of FsHA/FsCol beads: Cell attachment and cytotoxicity studies.Heliyon. 2023 May 1;9(5):e15838. doi: 10.1016/j.heliyon.2023.e15838. eCollection 2023 May. Heliyon. 2023. PMID: 37206015 Free PMC article.
-
Graphene in 3D Bioprinting.J Funct Biomater. 2024 Mar 25;15(4):82. doi: 10.3390/jfb15040082. J Funct Biomater. 2024. PMID: 38667539 Free PMC article. Review.
-
Effects of mechanical properties of carbon-based nanocomposites on scaffolds for tissue engineering applications: a comprehensive review.Nanoscale Adv. 2023 Dec 22;6(2):337-366. doi: 10.1039/d3na00554b. eCollection 2024 Jan 16. Nanoscale Adv. 2023. PMID: 38235087 Free PMC article. Review.
-
Metastable FeMg particles for controlling degradation rate, mechanical properties, and biocompatibility of Poly(l-lactic) acid (PLLA) for orthopedic applications.Heliyon. 2023 Nov 20;9(12):e22552. doi: 10.1016/j.heliyon.2023.e22552. eCollection 2023 Dec. Heliyon. 2023. PMID: 38107306 Free PMC article.
-
Graphene-Lined Porous Gelatin Glycidyl Methacrylate Hydrogels: Implications for Tissue Engineering.ACS Appl Nano Mater. 2021 Nov 26;4(11):12650-12662. doi: 10.1021/acsanm.1c03201. Epub 2021 Nov 10. ACS Appl Nano Mater. 2021. PMID: 35252778 Free PMC article.
References
-
- Ambekar RS, Kandasubramanian B. Progress in the advancement of porous biopolymer scaffold: tissue engineering application. Ind Eng Chem Res. 2019;58(16):6163–6194.
-
- Murphy SV, De Coppi P, Atala A. Opportunities and challenges of translational 3D bioprinting. Nat Biomed Eng. 2020;4(4):370–380. - PubMed
-
- Kaviyarasu K, Manikandan E, Kennedy J, Maaza M. Synthesis and analytical applications of photoluminescent carbon nanosheet by exfoliation of graphite oxide without purification. J Mater Sci-Mater El. 2016;27(12):13080–13085.
-
- Baradaran S, Moghaddam E, Basirun WJ, Mehrali M, Sookhakian M, Hamdi M, et al. Mechanical properties and biomedical applications of a nanotube hydroxyapatite-reduced graphene oxide composite. Carbon. 2014;69:32–45.
-
- Ramadas M, Bharath G, Ponpandian N, Ballamurugan AM. Investigation on biophysical properties of Hydroxyapatite/Graphene oxide (HAp/GO) based binary nanocomposite for biomedical applications. Mater Chem Phys. 2017;199:179–184.
Publication types
MeSH terms
Substances
LinkOut - more resources
Full Text Sources
Miscellaneous