The interactome of CLUH reveals its association to SPAG5 and its co-translational proximity to mitochondrial proteins
- PMID: 35012549
- PMCID: PMC8744257
- DOI: 10.1186/s12915-021-01213-y
The interactome of CLUH reveals its association to SPAG5 and its co-translational proximity to mitochondrial proteins
Abstract
Background: Mitochondria require thousands of proteins to fulfill their essential function in energy production and other fundamental biological processes. These proteins are mostly encoded by the nuclear genome, translated in the cytoplasm before being imported into the organelle. RNA binding proteins (RBPs) are central players in the regulation of this process by affecting mRNA translation, stability, or localization. CLUH is an RBP recognizing specifically mRNAs coding for mitochondrial proteins, but its precise molecular function and interacting partners remain undiscovered in mammals.
Results: Here we reveal for the first time CLUH interactome in mammalian cells. Using both co-IP and BioID proximity-labeling approaches, we identify novel molecular partners interacting stably or transiently with CLUH in HCT116 cells and mouse embryonic stem cells. We reveal stable RNA-independent interactions of CLUH with itself and with SPAG5 in cytosolic granular structures. More importantly, we uncover an unexpected proximity of CLUH to mitochondrial proteins and their cognate mRNAs in the cytosol. We show that this interaction occurs during the process of active translation and is dependent on CLUH TPR domain.
Conclusions: Overall, through the analysis of CLUH interactome, our study sheds a new light on CLUH molecular function by revealing new partners and by highlighting its link to the translation and subcellular localization of some mRNAs coding for mitochondrial proteins.
Keywords: BioID; CLUH; Localized translation; Nuclear encoded mitochondrial proteins; Proximity labeling; RNA binding proteins; SPAG5; Translation; TurboID.
© 2021. The Author(s).
Conflict of interest statement
The authors declare that they have no competing interests.
Figures
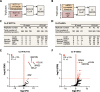
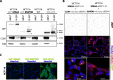
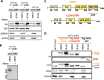
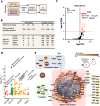
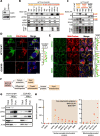
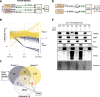
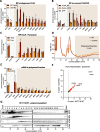
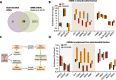
Similar articles
-
CLUH maintains functional mitochondria and translation in motoneuronal axons and prevents peripheral neuropathy.Sci Adv. 2024 May 31;10(22):eadn2050. doi: 10.1126/sciadv.adn2050. Epub 2024 May 29. Sci Adv. 2024. PMID: 38809982 Free PMC article.
-
Characterization of Factors Involved in Localized Translation Near Mitochondria by Ribosome-Proximity Labeling.Front Cell Dev Biol. 2019 Nov 26;7:305. doi: 10.3389/fcell.2019.00305. eCollection 2019. Front Cell Dev Biol. 2019. PMID: 31929983 Free PMC article.
-
CLUH regulates mitochondrial biogenesis by binding mRNAs of nuclear-encoded mitochondrial proteins.J Cell Biol. 2014 Oct 27;207(2):213-23. doi: 10.1083/jcb.201403129. J Cell Biol. 2014. PMID: 25349259 Free PMC article.
-
A concert of RNA-binding proteins coordinates mitochondrial function.Crit Rev Biochem Mol Biol. 2018 Dec;53(6):652-666. doi: 10.1080/10409238.2018.1553927. Crit Rev Biochem Mol Biol. 2018. PMID: 30741581 Review.
-
Localization of Nuclear-Encoded mRNAs to Mitochondria Outer Surface.Biochemistry (Mosc). 2016 Oct;81(10):1038-1043. doi: 10.1134/S0006297916100023. Biochemistry (Mosc). 2016. PMID: 27908229 Review.
Cited by
-
CLUH controls astrin-1 expression to couple mitochondrial metabolism to cell cycle progression.Elife. 2022 May 13;11:e74552. doi: 10.7554/eLife.74552. Elife. 2022. PMID: 35559794 Free PMC article.
-
CLUH maintains functional mitochondria and translation in motoneuronal axons and prevents peripheral neuropathy.Sci Adv. 2024 May 31;10(22):eadn2050. doi: 10.1126/sciadv.adn2050. Epub 2024 May 29. Sci Adv. 2024. PMID: 38809982 Free PMC article.
-
FRIENDLY (FMT) is an RNA binding protein associated with cytosolic ribosomes at the mitochondrial surface.Plant J. 2022 Oct;112(2):309-321. doi: 10.1111/tpj.15962. Epub 2022 Sep 12. Plant J. 2022. PMID: 36050837 Free PMC article.
-
Loss of Drosophila Clueless differentially affects the mitochondrial proteome compared to loss of Sod2 and Pink1.Front Physiol. 2022 Oct 26;13:1004099. doi: 10.3389/fphys.2022.1004099. eCollection 2022. Front Physiol. 2022. PMID: 36388112 Free PMC article.
-
Rummagene: massive mining of gene sets from supporting materials of biomedical research publications.Commun Biol. 2024 Apr 20;7(1):482. doi: 10.1038/s42003-024-06177-7. Commun Biol. 2024. PMID: 38643247 Free PMC article.
References
Publication types
MeSH terms
Substances
LinkOut - more resources
Full Text Sources
Molecular Biology Databases