3D-bioprinted peptide coupling patches for wound healing
- PMID: 34977527
- PMCID: PMC8683759
- DOI: 10.1016/j.mtbio.2021.100188
3D-bioprinted peptide coupling patches for wound healing
Abstract
Chronic wounds caused by severe trauma remain a serious challenge for clinical treatment. In this study, we developed a novel angiogenic 3D-bioprinted peptide patch to improve skin wound healing. The 3D-bioprinted technology can fabricate individual patches according to the shape characteristics of the damaged tissue. Gelatin methacryloyl (GelMA) and hyaluronic acid methacryloyl (HAMA) have excellent biocompatibility and biodegradability, and were used as a biomaterial to produce bioprinted patches. The pro-angiogenic QHREDGS peptide was covalently conjugated to the 3D-bioprinted GelMA/HAMA patches, extending the release of QHREDGS and improving the angiogenic properties of the patch. Our results demonstrated that these 3D-bioprinted peptide patches showed excellent biocompatibility, angiogenesis, and tissue repair both in vivo and in vitro. These findings indicated that 3D-bioprinted peptide patches improved skin wound healing and could be used in other tissue engineering applications.
Keywords: 3D-printing; Angiogenesis; Gelatin methacryloyl (GelMA); Hyaluronic acid methacryloyl (HAMA); Peptide; Wound healing.
© 2021 The Authors.
Conflict of interest statement
The authors declare that they have no known competing financial interests or personal relationships that could have appeared to influence the work reported in this paper.
Figures
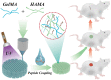
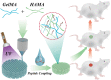
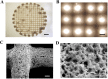
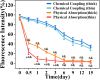
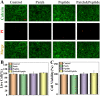
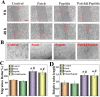
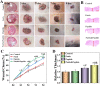
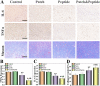
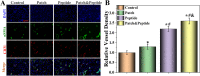
Similar articles
-
3D-bioprinted double-crosslinked angiogenic alginate/chondroitin sulfate patch for diabetic wound healing.Int J Biol Macromol. 2023 May 1;236:123952. doi: 10.1016/j.ijbiomac.2023.123952. Epub 2023 Mar 8. Int J Biol Macromol. 2023. PMID: 36894059
-
Application of 3D-printed tissue-engineered skin substitute using innovative biomaterial loaded with human adipose-derived stem cells in wound healing.Int J Bioprint. 2023 Jan 31;9(2):674. doi: 10.18063/ijb.v9i2.674. eCollection 2023. Int J Bioprint. 2023. PMID: 37065662 Free PMC article.
-
Biomaterial composition and stiffness as decisive properties of 3D bioprinted constructs for type II collagen stimulation.Acta Biomater. 2022 Oct 15;152:221-234. doi: 10.1016/j.actbio.2022.08.058. Epub 2022 Aug 29. Acta Biomater. 2022. PMID: 36049623
-
Recent Advances on Bioprinted Gelatin Methacrylate-Based Hydrogels for Tissue Repair.Tissue Eng Part A. 2021 Jun;27(11-12):679-702. doi: 10.1089/ten.TEA.2020.0350. Epub 2021 Mar 9. Tissue Eng Part A. 2021. PMID: 33499750 Review.
-
Recent Advances in the Design of Three-Dimensional and Bioprinted Scaffolds for Full-Thickness Wound Healing.Tissue Eng Part B Rev. 2022 Feb;28(1):160-181. doi: 10.1089/ten.TEB.2020.0339. Epub 2021 Feb 22. Tissue Eng Part B Rev. 2022. PMID: 33446047 Review.
Cited by
-
Progress in the application of sustained-release drug microspheres in tissue engineering.Mater Today Bio. 2022 Aug 13;16:100394. doi: 10.1016/j.mtbio.2022.100394. eCollection 2022 Dec. Mater Today Bio. 2022. PMID: 36042853 Free PMC article. Review.
-
Innovative Treatment Strategies to Accelerate Wound Healing: Trajectory and Recent Advancements.Cells. 2022 Aug 6;11(15):2439. doi: 10.3390/cells11152439. Cells. 2022. PMID: 35954282 Free PMC article. Review.
-
Current Trends on Innovative Technologies in Topical Wound Care for Advanced Healing and Management.Curr Drug Res Rev. 2024;16(3):319-332. doi: 10.2174/0125899775262048230925054922. Curr Drug Res Rev. 2024. PMID: 37807417 Review.
-
Recent advances on 3D-bioprinted gelatin methacrylate hydrogels for tissue engineering in wound healing: A review of current applications and future prospects.Int Wound J. 2024 Apr;21(4):e14533. doi: 10.1111/iwj.14533. Epub 2023 Dec 9. Int Wound J. 2024. PMID: 38069620 Free PMC article. Review.
-
Gelatin Methacrylate Hydrogel for Tissue Engineering Applications-A Review on Material Modifications.Pharmaceuticals (Basel). 2022 Jan 29;15(2):171. doi: 10.3390/ph15020171. Pharmaceuticals (Basel). 2022. PMID: 35215284 Free PMC article. Review.
References
-
- MacNeil Sheila. Progress and opportunities for tissue-engineered skin. Nature. 2007;445(7130):874–880. - PubMed
-
- Fife C.E., Carter M.J., Walker D., Thomson B. Wound care outcomes and associated cost among patients treated in us outpatient wound centers: data from the us wound registry. Wounds. 2012;24(1):10–17. - PubMed
LinkOut - more resources
Full Text Sources