Tryptophan Production Maximization in a Fed-Batch Bioreactor with Modified E. coli Cells, by Optimizing Its Operating Policy Based on an Extended Structured Cell Kinetic Model
- PMID: 34940363
- PMCID: PMC8698263
- DOI: 10.3390/bioengineering8120210
Tryptophan Production Maximization in a Fed-Batch Bioreactor with Modified E. coli Cells, by Optimizing Its Operating Policy Based on an Extended Structured Cell Kinetic Model
Abstract
Hybrid kinetic models, linking structured cell metabolic processes to the dynamics of macroscopic variables of the bioreactor, are more and more used in engineering evaluations to derive more precise predictions of the process dynamics under variable operating conditions. Depending on the cell model complexity, such a math tool can be used to evaluate the metabolic fluxes in relation to the bioreactor operating conditions, thus suggesting ways to genetically modify the microorganism for certain purposes. Even if development of such an extended dynamic model requires more experimental and computational efforts, its use is advantageous. The approached probative example refers to a model simulating the dynamics of nanoscale variables from several pathways of the central carbon metabolism (CCM) of Escherichia coli cells, linked to the macroscopic state variables of a fed-batch bioreactor (FBR) used for the tryptophan (TRP) production. The used E. coli strain was modified to replace the PTS system for glucose (GLC) uptake with a more efficient one. The study presents multiple elements of novelty: (i) the experimentally validated modular model itself, and (ii) its efficiency in computationally deriving an optimal operation policy of the FBR.
Keywords: cell structured kinetic model; fed-batch bioreactor optimization; glycolysis; hybrid modular model; modified E. coli; tryptophan synthesis.
Conflict of interest statement
The authors declare that they have no known competing financial interests or personal relationships that could have appeared to influence the work reported in this paper. The authors confirm that his paper has no conflict of interest of any kind and of any nature. While the advice and the information in this work are believed to be true and accurate at the date of publication, the entire responsibility for the work content lies with the authors. The authors’ institutions have no responsibility for the content of this work.
Figures
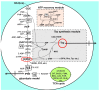
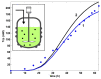
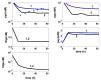
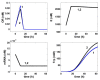
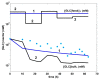
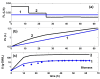
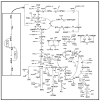
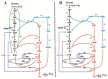
Similar articles
-
In silico Determination of Some Conditions Leading to Glycolytic Oscillations and Their Interference With Some Other Processes in E. coli Cells.Front Chem. 2020 Oct 28;8:526679. doi: 10.3389/fchem.2020.526679. eCollection 2020. Front Chem. 2020. PMID: 33195042 Free PMC article.
-
Model-Based Optimization of a Fed-Batch Bioreactor for mAb Production Using a Hybridoma Cell Culture.Molecules. 2020 Nov 30;25(23):5648. doi: 10.3390/molecules25235648. Molecules. 2020. PMID: 33266156 Free PMC article.
-
Discovery of feed-forward regulation in L-tryptophan biosynthesis and its use in metabolic engineering of E. coli for efficient tryptophan bioproduction.Metab Eng. 2018 May;47:434-444. doi: 10.1016/j.ymben.2018.05.001. Epub 2018 May 5. Metab Eng. 2018. PMID: 29733896
-
Alteration of the biochemical valves in the central metabolism of Escherichia coli.Ann N Y Acad Sci. 1994 Nov 30;745:21-34. doi: 10.1111/j.1749-6632.1994.tb44361.x. Ann N Y Acad Sci. 1994. PMID: 7832509 Review.
-
Microbial production of polyhydroxybutyrate with tailor-made properties: an integrated modelling approach and experimental validation.Biotechnol Adv. 2012 Jan-Feb;30(1):329-37. doi: 10.1016/j.biotechadv.2011.06.021. Epub 2011 Jul 5. Biotechnol Adv. 2012. PMID: 21756991 Review.
References
-
- Ghose T.K., Fiechter A., Blakebrough N. Advances in Biochemical Engineering. Volume 7–10 Springer; Berlin, Germany: 1977.
-
- Moser A. Bioprocess Technology—Kinetics and Reactors. Springer; Berlin, Germany: 1988.
-
- Maria G., Peptănaru I.M. Model-based optimization of mannitol production by using a sequence of batch reactors for a coupled bi-enzymatic process—A dynamic approach. Dynamics. 2021;1:8. doi: 10.3390/dynamics1010008. - DOI
-
- Liese A., Seelbach K., Wandrey C. Industrial Biotransformations. Wiley-VCH; Weinheim, Germany: 2006.
LinkOut - more resources
Full Text Sources