Dynamic and cell-specific transport networks for intracellular copper ions
- PMID: 34734631
- PMCID: PMC8627558
- DOI: 10.1242/jcs.240523
Dynamic and cell-specific transport networks for intracellular copper ions
Abstract
Copper (Cu) homeostasis is essential for the development and function of many organisms. In humans, Cu misbalance causes serious pathologies and has been observed in a growing number of diseases. This Review focuses on mammalian Cu(I) transporters and highlights recent studies on regulation of intracellular Cu fluxes. Cu is used by essential metabolic enzymes for their activity. These enzymes are located in various intracellular compartments and outside cells. When cells differentiate, or their metabolic state is otherwise altered, the need for Cu in different cell compartments change, and Cu has to be redistributed to accommodate these changes. The Cu transporters SLC31A1 (CTR1), SLC31A2 (CTR2), ATP7A and ATP7B regulate Cu content in cellular compartments and maintain Cu homeostasis. Increasing numbers of regulatory proteins have been shown to contribute to multifaceted regulation of these Cu transporters. It is becoming abundantly clear that the Cu transport networks are dynamic and cell specific. The comparison of the Cu transport machinery in the liver and intestine illustrates the distinct composition and dissimilar regulatory response of their Cu transporters to changing Cu levels.
Keywords: ATP7A; ATP7B; Copper; SLC31A1; SLC31A2; Transport.
© 2021. Published by The Company of Biologists Ltd.
Conflict of interest statement
Competing interests I declare no competing or financial interests.
Figures
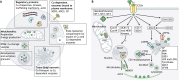
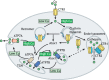
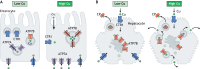
Similar articles
-
ATP7A and ATP7B copper transporters have distinct functions in the regulation of neuronal dopamine-β-hydroxylase.J Biol Chem. 2018 Dec 28;293(52):20085-20098. doi: 10.1074/jbc.RA118.004889. Epub 2018 Oct 19. J Biol Chem. 2018. PMID: 30341172 Free PMC article.
-
Involvement of CTR1 and ATP7A in lead (Pb)-induced copper (Cu) accumulation in choroidal epithelial cells.Toxicol Lett. 2014 Feb 10;225(1):110-8. doi: 10.1016/j.toxlet.2013.11.034. Epub 2013 Dec 6. Toxicol Lett. 2014. PMID: 24316150 Free PMC article.
-
The mammalian copper transporters CTR1 and CTR2 and their roles in development and disease.Int J Biochem Cell Biol. 2013 May;45(5):960-3. doi: 10.1016/j.biocel.2013.01.018. Epub 2013 Feb 4. Int J Biochem Cell Biol. 2013. PMID: 23391749 Review.
-
[Structure and function of ATP7A and ATP7B proteins--Cu-transporting ATPases].Postepy Biochem. 2010;56(3):317-27. Postepy Biochem. 2010. PMID: 21117320 Review. Polish.
-
Function and regulation of human copper-transporting ATPases.Physiol Rev. 2007 Jul;87(3):1011-46. doi: 10.1152/physrev.00004.2006. Physiol Rev. 2007. PMID: 17615395 Review.
Cited by
-
Comprehensive analysis of cuproptosis-related long noncoding RNA for predicting prognostic and diagnostic value and immune landscape in colorectal adenocarcinoma.Hum Genomics. 2023 Mar 13;17(1):22. doi: 10.1186/s40246-023-00469-5. Hum Genomics. 2023. PMID: 36915193 Free PMC article.
-
Cuproptosis: mechanisms and links with cancers.Mol Cancer. 2023 Mar 7;22(1):46. doi: 10.1186/s12943-023-01732-y. Mol Cancer. 2023. PMID: 36882769 Free PMC article. Review.
-
Mechanism of Cu entry into the brain: many unanswered questions.Neural Regen Res. 2024 Nov 1;19(11):2421-2429. doi: 10.4103/1673-5374.393107. Epub 2024 Jan 8. Neural Regen Res. 2024. PMID: 38526278 Free PMC article.
-
A ceRNA network-mediated over-expression of cuproptosis-related gene SLC31A1 correlates with poor prognosis and positive immune infiltration in breast cancer.Front Med (Lausanne). 2023 May 18;10:1194046. doi: 10.3389/fmed.2023.1194046. eCollection 2023. Front Med (Lausanne). 2023. PMID: 37275369 Free PMC article.
-
Sleep fragmentation exacerbates myocardial ischemia‒reperfusion injury by promoting copper overload in cardiomyocytes.Nat Commun. 2024 May 7;15(1):3834. doi: 10.1038/s41467-024-48227-y. Nat Commun. 2024. PMID: 38714741 Free PMC article.
References
-
- Ashino, T., Sudhahar, V., Urao, N., Oshikawa, J., Chen, G.-F., Wang, H., Huo, Y., Finney, L., Vogt, S., McKinney, R. D.et al. (2010). Unexpected role of the copper transporter ATP7A in PDGF-induced vascular smooth muscle cell migration. Circ. Res. 107, 787-799. 10.1161/CIRCRESAHA.110.225334 - DOI - PMC - PubMed
-
- Baguña Torres, J., Yu, Z., Bordoloi, J., Sunassee, K., Smith, D., Smith, C., Chen, O., Purchase, R., Tuschl, K., Spencer, J.et al. (2019). Imaging of changes in copper trafficking and redistribution in a mouse model of Niemann-Pick C disease using positron emission tomography. Biometals 32, 293-306. 10.1007/s10534-019-00185-5 - DOI - PMC - PubMed
-
- Baker, Z. N., Jett, K., Boulet, A., Hossain, A., Cobine, P. A., Kim, B.-E., El Zawily, A. M., Lee, L., Tibbits, G. F., Petris, M. J.et al. (2017b). The mitochondrial metallochaperone SCO1 maintains CTR1 at the plasma membrane to preserve copper homeostasis in the murine heart. Hum. Mol. Genet. 26, 4617-4628. 10.1093/hmg/ddx344 - DOI - PMC - PubMed
Publication types
MeSH terms
Substances
Grants and funding
LinkOut - more resources
Full Text Sources
Research Materials