Development of astrocyte morphology and function in mouse visual thalamus
- PMID: 34636034
- PMCID: PMC8957486
- DOI: 10.1002/cne.25261
Development of astrocyte morphology and function in mouse visual thalamus
Abstract
The rodent visual thalamus has served as a powerful model to elucidate the cellular and molecular mechanisms that underlie sensory circuit formation and function. Despite significant advances in our understanding of the role of axon-target interactions and neural activity in orchestrating circuit formation in visual thalamus, the role of non-neuronal cells, such as astrocytes, is less clear. In fact, we know little about the transcriptional identity and development of astrocytes in mouse visual thalamus. To address this gap in knowledge, we studied the expression of canonical astrocyte molecules in visual thalamus using immunostaining, in situ hybridization, and reporter lines. While our data suggests some level of heterogeneity of astrocytes in different nuclei of the visual thalamus, the majority of thalamic astrocytes appeared to be labeled in Aldh1l1-EGFP mice. This led us to use this transgenic line to characterize the neonatal and postnatal development of these cells in visual thalamus. Our data show that not only have the entire cohort of astrocytes migrated into visual thalamus by eye-opening but they also have acquired their adult-like morphology, even while retinogeniculate synapses are still maturing. Furthermore, ultrastructural, immunohistochemical, and functional approaches revealed that by eye-opening, thalamic astrocytes ensheathe retinogeniculate synapses and are capable of efficient uptake of glutamate. Taken together, our results reveal that the morphological, anatomical, and functional development of astrocytes in visual thalamus occurs prior to eye-opening and the emergence of experience-dependent visual activity.
Keywords: astrocytes; dorsal lateral geniculate nucleus; retinogeniculate synapse; thalamus; ventral lateral geniculate nucleus.
© 2021 Wiley Periodicals LLC.
Conflict of interest statement
Figures
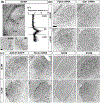
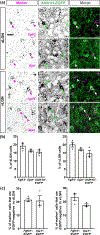
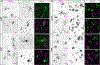
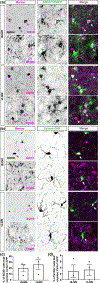
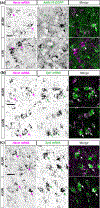
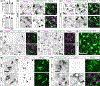
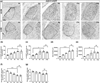
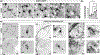
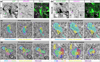
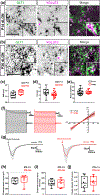
Similar articles
-
Nuclei-specific differences in nerve terminal distribution, morphology, and development in mouse visual thalamus.Neural Dev. 2014 Jul 10;9:16. doi: 10.1186/1749-8104-9-16. Neural Dev. 2014. PMID: 25011644 Free PMC article.
-
Organization, Function, and Development of the Mouse Retinogeniculate Synapse.Annu Rev Vis Sci. 2020 Sep 15;6:261-285. doi: 10.1146/annurev-vision-121219-081753. Annu Rev Vis Sci. 2020. PMID: 32936733 Review.
-
Changes in input strength and number are driven by distinct mechanisms at the retinogeniculate synapse.J Neurophysiol. 2014 Aug 15;112(4):942-50. doi: 10.1152/jn.00175.2014. Epub 2014 May 21. J Neurophysiol. 2014. PMID: 24848465 Free PMC article.
-
Development of the mammalian retinogeniculate pathway: target finding, transient synapses and binocular segregation.J Exp Biol. 1990 Oct;153:85-104. doi: 10.1242/jeb.153.1.85. J Exp Biol. 1990. PMID: 2280230 Review.
-
The Metabotropic Glutamate Receptor Subtype 1 Mediates Experience-Dependent Maintenance of Mature Synaptic Connectivity in the Visual Thalamus.Neuron. 2016 Sep 7;91(5):1097-1109. doi: 10.1016/j.neuron.2016.07.035. Epub 2016 Aug 18. Neuron. 2016. PMID: 27545713
Cited by
-
Astrocytes require perineuronal nets to maintain synaptic homeostasis in mice.Nat Neurosci. 2024 Aug;27(8):1475-1488. doi: 10.1038/s41593-024-01714-3. Epub 2024 Jul 17. Nat Neurosci. 2024. PMID: 39020018 Free PMC article.
-
Sonic hedgehog-dependent recruitment of GABAergic interneurons into the developing visual thalamus.Elife. 2022 Nov 7;11:e79833. doi: 10.7554/eLife.79833. Elife. 2022. PMID: 36342840 Free PMC article.
-
Glia Regulate the Development, Function, and Plasticity of the Visual System From Retina to Cortex.Front Neural Circuits. 2022 Feb 1;16:826664. doi: 10.3389/fncir.2022.826664. eCollection 2022. Front Neural Circuits. 2022. PMID: 35177968 Free PMC article. Review.
-
A glial perspective on the extracellular matrix and perineuronal net remodeling in the central nervous system.Front Cell Neurosci. 2022 Oct 20;16:1022754. doi: 10.3389/fncel.2022.1022754. eCollection 2022. Front Cell Neurosci. 2022. PMID: 36339816 Free PMC article. Review.
-
Post-Injury Buprenorphine Administration Is Associated with Long-Term Region-Specific Glial Alterations in Rats.Pharmaceutics. 2022 Sep 28;14(10):2068. doi: 10.3390/pharmaceutics14102068. Pharmaceutics. 2022. PMID: 36297504 Free PMC article.
References
-
- Bayraktar OA, Bartels T, Holmqvist S, Kleshchevnikov V, Martirosyan A, Polioudakis D, Ben Haim L, Young AMH, Batiuk MY, Prakash K, Brown A, Roberts K, Paredes MF, Kawaguchi R, Stockley JH, Sabeur K, Chang SM, Huang E, Hutchinson P, … Rowitch DH (2020). Astrocyte layers in the mammalian cerebral cortex revealed by a single-cell in situ transcriptomic map. Nature Neuroscience, 23(4), 500–509. 10.1038/s41593-020-0602-1 - DOI - PMC - PubMed
Publication types
MeSH terms
Grants and funding
LinkOut - more resources
Full Text Sources
Molecular Biology Databases
Miscellaneous