Paired heavy- and light-chain signatures contribute to potent SARS-CoV-2 neutralization in public antibody responses
- PMID: 34587480
- PMCID: PMC8479507
- DOI: 10.1016/j.celrep.2021.109771
Paired heavy- and light-chain signatures contribute to potent SARS-CoV-2 neutralization in public antibody responses
Abstract
Understanding mechanisms of protective antibody recognition can inform vaccine and therapeutic strategies against SARS-CoV-2. We report a monoclonal antibody, 910-30, targeting the SARS-CoV-2 receptor-binding site for ACE2 as a member of a public antibody response encoded by IGHV3-53/IGHV3-66 genes. Sequence and structural analyses of 910-30 and related antibodies explore how class recognition features correlate with SARS-CoV-2 neutralization. Cryo-EM structures of 910-30 bound to the SARS-CoV-2 spike trimer reveal binding interactions and its ability to disassemble spike. Despite heavy-chain sequence similarity, biophysical analyses of IGHV3-53/3-66-encoded antibodies highlight the importance of native heavy:light pairings for ACE2-binding competition and SARS-CoV-2 neutralization. We develop paired heavy:light class sequence signatures and determine antibody precursor prevalence to be ∼1 in 44,000 human B cells, consistent with public antibody identification in several convalescent COVID-19 patients. These class signatures reveal genetic, structural, and functional immune features that are helpful in accelerating antibody-based medical interventions for SARS-CoV-2.
Keywords: B-cell; SARS-CoV-2; biotechnology; immunity; neutralization; public antibody; virology; yeast display.
Copyright © 2021 The Author(s). Published by Elsevier Inc. All rights reserved.
Conflict of interest statement
Declaration of interests B.B.B., A.S.F., M.O., P.W., L.L., S.N.L.A., J.R.W., X.P., B.M., D.D.H., and B.J.D. declare competing financial interests in the form of a provisional patent application filed by the University of Kansas.
Figures
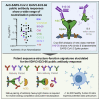
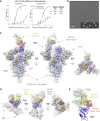
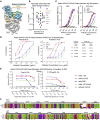
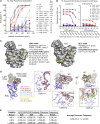
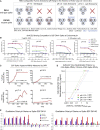
Update of
-
Paired heavy and light chain signatures contribute to potent SARS-CoV-2 neutralization in public antibody responses.bioRxiv [Preprint]. 2021 Jan 3:2020.12.31.424987. doi: 10.1101/2020.12.31.424987. bioRxiv. 2021. Update in: Cell Rep. 2021 Oct 5;37(1):109771. doi: 10.1016/j.celrep.2021.109771. PMID: 33442681 Free PMC article. Updated. Preprint.
Similar articles
-
Structural basis for accommodation of emerging B.1.351 and B.1.1.7 variants by two potent SARS-CoV-2 neutralizing antibodies.Structure. 2021 Jul 1;29(7):655-663.e4. doi: 10.1016/j.str.2021.05.014. Epub 2021 Jun 9. Structure. 2021. PMID: 34111408 Free PMC article.
-
Potent neutralization of SARS-CoV-2 variants of concern by an antibody with an uncommon genetic signature and structural mode of spike recognition.Cell Rep. 2021 Oct 5;37(1):109784. doi: 10.1016/j.celrep.2021.109784. Epub 2021 Sep 16. Cell Rep. 2021. PMID: 34592170 Free PMC article.
-
Paired heavy and light chain signatures contribute to potent SARS-CoV-2 neutralization in public antibody responses.bioRxiv [Preprint]. 2021 Jan 3:2020.12.31.424987. doi: 10.1101/2020.12.31.424987. bioRxiv. 2021. Update in: Cell Rep. 2021 Oct 5;37(1):109771. doi: 10.1016/j.celrep.2021.109771. PMID: 33442681 Free PMC article. Updated. Preprint.
-
Recognition of the SARS-CoV-2 receptor binding domain by neutralizing antibodies.Biochem Biophys Res Commun. 2021 Jan 29;538:192-203. doi: 10.1016/j.bbrc.2020.10.012. Epub 2020 Oct 10. Biochem Biophys Res Commun. 2021. PMID: 33069360 Free PMC article. Review.
-
Analysis of the molecular mechanism of SARS-CoV-2 antibodies.Biochem Biophys Res Commun. 2021 Aug 20;566:45-52. doi: 10.1016/j.bbrc.2021.06.001. Epub 2021 Jun 5. Biochem Biophys Res Commun. 2021. PMID: 34116356 Free PMC article. Review.
Cited by
-
Increased Resistance of SARS-CoV-2 Variants B.1.351 and B.1.1.7 to Antibody Neutralization.Res Sq [Preprint]. 2021 Jan 29:rs.3.rs-155394. doi: 10.21203/rs.3.rs-155394/v1. Res Sq. 2021. Update in: Nature. 2021 May;593(7857):130-135. doi: 10.1038/s41586-021-03398-2. PMID: 33532763 Free PMC article. Updated. Preprint.
-
Structural and functional ramifications of antigenic drift in recent SARS-CoV-2 variants.bioRxiv [Preprint]. 2021 Feb 17:2021.02.16.430500. doi: 10.1101/2021.02.16.430500. bioRxiv. 2021. Update in: Science. 2021 Aug 13;373(6556):818-823. doi: 10.1126/science.abh1139. PMID: 33619487 Free PMC article. Updated. Preprint.
-
Structural basis for accommodation of emerging B.1.351 and B.1.1.7 variants by two potent SARS-CoV-2 neutralizing antibodies.Structure. 2021 Jul 1;29(7):655-663.e4. doi: 10.1016/j.str.2021.05.014. Epub 2021 Jun 9. Structure. 2021. PMID: 34111408 Free PMC article.
-
Stabilization of the SARS-CoV-2 receptor binding domain by protein core redesign and deep mutational scanning.Protein Eng Des Sel. 2022 Feb 17;35:gzac002. doi: 10.1093/protein/gzac002. Protein Eng Des Sel. 2022. PMID: 35325236 Free PMC article.
-
Molecular probes of spike ectodomain and its subdomains for SARS-CoV-2 variants, Alpha through Omicron.bioRxiv [Preprint]. 2021 Dec 30:2021.12.29.474491. doi: 10.1101/2021.12.29.474491. bioRxiv. 2021. Update in: PLoS One. 2022 May 24;17(5):e0268767. doi: 10.1371/journal.pone.0268767. PMID: 35018379 Free PMC article. Updated. Preprint.
References
-
- Adams P.D., Gopal K., Grosse-Kunstleve R.W., Hung L.-W., Ioerger T.R., McCoy A.J., Moriarty N.W., Pai R.K., Read R.J., Romo T.D., et al. Recent developments in the PHENIX software for automated crystallographic structure determination. J. Synchrotron Radiat. 2004;11:53–55. - PubMed
-
- Barnes C.O., West A.P., Jr., Huey-Tubman K.E., Hoffmann M.A.G., Sharaf N.G., Hoffman P.R., Koranda N., Gristick H.B., Gaebler C., Muecksch F., et al. Structures of human antibodies bound to SARS-CoV-2 spike reveal common epitopes and recurrent features of antibodies. Cell. 2020;182:828–842.e16. - PMC - PubMed
-
- Benatuil L., Perez J.M., Belk J., Hsieh C.-M. An improved yeast transformation method for the generation of very large human antibody libraries. Protein Eng. Des. Sel. 2010;23:155–159. - PubMed
Publication types
MeSH terms
Substances
Grants and funding
LinkOut - more resources
Full Text Sources
Other Literature Sources
Medical
Miscellaneous