Screening by deep sequencing reveals mediators of microRNA tailing in C. elegans
- PMID: 34586415
- PMCID: PMC8565341
- DOI: 10.1093/nar/gkab840
Screening by deep sequencing reveals mediators of microRNA tailing in C. elegans
Abstract
microRNAs are frequently modified by addition of untemplated nucleotides to the 3' end, but the role of this tailing is often unclear. Here we characterize the prevalence and functional consequences of microRNA tailing in vivo, using Caenorhabditis elegans. MicroRNA tailing in C. elegans consists mostly of mono-uridylation of mature microRNA species, with rarer mono-adenylation which is likely added to microRNA precursors. Through a targeted RNAi screen, we discover that the TUT4/TUT7 gene family member CID-1/CDE-1/PUP-1 is required for uridylation, whereas the GLD2 gene family member F31C3.2-here named GLD-2-related 2 (GLDR-2)-is required for adenylation. Thus, the TUT4/TUT7 and GLD2 gene families have broadly conserved roles in miRNA modification. We specifically examine the role of tailing in microRNA turnover. We determine half-lives of microRNAs after acute inactivation of microRNA biogenesis, revealing that half-lives are generally long (median = 20.7 h), as observed in other systems. Although we observe that the proportion of tailed species increases over time after biogenesis, disrupting tailing does not alter microRNA decay. Thus, tailing is not a global regulator of decay in C. elegans. Nonetheless, by identifying the responsible enzymes, this study lays the groundwork to explore whether tailing plays more specialized context- or miRNA-specific regulatory roles.
Published by Oxford University Press on behalf of Nucleic Acids Research 2021.
Figures
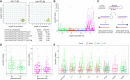
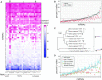
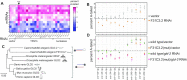

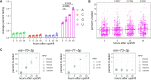
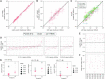
Similar articles
-
TENT2, TUT4, and TUT7 selectively regulate miRNA sequence and abundance.Nat Commun. 2022 Sep 7;13(1):5260. doi: 10.1038/s41467-022-32969-8. Nat Commun. 2022. PMID: 36071058 Free PMC article.
-
TUT7 controls the fate of precursor microRNAs by using three different uridylation mechanisms.EMBO J. 2015 Jul 2;34(13):1801-15. doi: 10.15252/embj.201590931. Epub 2015 May 15. EMBO J. 2015. PMID: 25979828 Free PMC article.
-
Active turnover modulates mature microRNA activity in Caenorhabditis elegans.Nature. 2009 Sep 24;461(7263):546-9. doi: 10.1038/nature08349. Epub 2009 Sep 6. Nature. 2009. PMID: 19734881
-
Roles of microRNAs in the Caenorhabditis elegans nervous system.J Genet Genomics. 2013 Sep 20;40(9):445-52. doi: 10.1016/j.jgg.2013.07.002. Epub 2013 Aug 7. J Genet Genomics. 2013. PMID: 24053946 Review.
-
Analysis of microRNA expression and function.Methods Cell Biol. 2011;106:219-252. doi: 10.1016/B978-0-12-544172-8.00008-6. Methods Cell Biol. 2011. PMID: 22118279 Free PMC article. Review.
Cited by
-
Recent advances in understanding microRNA function and regulation in C. elegans.Semin Cell Dev Biol. 2024 Feb 15;154(Pt A):4-13. doi: 10.1016/j.semcdb.2023.03.011. Epub 2023 Apr 11. Semin Cell Dev Biol. 2024. PMID: 37055330 Review.
-
TENT2, TUT4, and TUT7 selectively regulate miRNA sequence and abundance.Nat Commun. 2022 Sep 7;13(1):5260. doi: 10.1038/s41467-022-32969-8. Nat Commun. 2022. PMID: 36071058 Free PMC article.
-
Catalytic residues of microRNA Argonautes play a modest role in microRNA star strand destabilization in C. elegans.Nucleic Acids Res. 2024 May 22;52(9):4985-5001. doi: 10.1093/nar/gkae170. Nucleic Acids Res. 2024. PMID: 38471816 Free PMC article.
-
Global profiling and annotation of templated isomiRs dynamics across Caenorhabditis elegans development.RNA Biol. 2022 Jan;19(1):928-942. doi: 10.1080/15476286.2022.2099646. RNA Biol. 2022. PMID: 35848953 Free PMC article.
-
What goes up must come down: off switches for regulatory RNAs.Genes Dev. 2024 Aug 20;38(13-14):597-613. doi: 10.1101/gad.351934.124. Genes Dev. 2024. PMID: 39111824 Free PMC article. Review.
References
Publication types
MeSH terms
Substances
Grants and funding
LinkOut - more resources
Full Text Sources
Molecular Biology Databases
Research Materials