Candida auris Cell Wall Mannosylation Contributes to Neutrophil Evasion through Pathways Divergent from Candida albicans and Candida glabrata
- PMID: 34160238
- PMCID: PMC8265655
- DOI: 10.1128/mSphere.00406-21
Candida auris Cell Wall Mannosylation Contributes to Neutrophil Evasion through Pathways Divergent from Candida albicans and Candida glabrata
Abstract
Candida auris, a recently emergent fungal pathogen, has caused invasive infections in health care settings worldwide. Mortality rates approach 60% and hospital spread poses a public health threat. Compared to other Candida spp., C. auris avoids triggering the antifungal activity of neutrophils, innate immune cells that are critical for responding to many invasive fungal infections, including candidiasis. However, the mechanism underpinning this immune evasion has been largely unknown. Here, we show that C. auris cell wall mannosylation contributes to the evasion of neutrophils ex vivo and in a zebrafish infection model. Genetic disruption of mannosylation pathways (PMR1 and VAN1) diminishes the outer cell wall mannan, unmasks immunostimulatory components, and promotes neutrophil engagement, phagocytosis, and killing. Upon examination of these pathways in other Candida spp. (Candida albicans and Candida glabrata), we did not find an impact on neutrophil interactions. These studies show how C. auris mannosylation contributes to neutrophil evasion though pathways distinct from other common Candida spp. The findings shed light on innate immune evasion for this emerging pathogen. IMPORTANCE The emerging fungal pathogen Candida auris presents a global public health threat. Therapeutic options are often limited for this frequently drug-resistant pathogen, and mortality rates for invasive disease are high. Previous study has demonstrated that neutrophils, leukocytes critical for the antifungal host defense, do not efficiently recognize and kill C. auris. Here, we show how the outer cell wall of C. auris promotes immune evasion. Disruption of this mannan polysaccharide layer renders C. auris susceptible to neutrophil killing ex vivo and in a zebrafish model of invasive candidiasis. The role of these mannosylation pathways for neutrophil evasion appears divergent from other common Candida species.
Keywords: Candida; Candida auris; Rac2; cell wall; glucan masking; immune evasion; innate immunity; mannan; neutrophil.
Figures
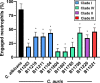
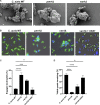
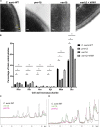
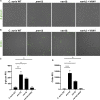
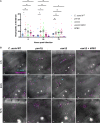
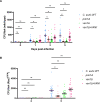
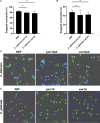
Similar articles
-
Emerging Fungal Pathogen Candida auris Evades Neutrophil Attack.mBio. 2018 Aug 21;9(4):e01403-18. doi: 10.1128/mBio.01403-18. mBio. 2018. PMID: 30131360 Free PMC article.
-
Evasion of Immune Surveillance in Low Oxygen Environments Enhances Candida albicans Virulence.mBio. 2018 Nov 6;9(6):e02120-18. doi: 10.1128/mBio.02120-18. mBio. 2018. PMID: 30401781 Free PMC article.
-
Innate immune response to Candida auris.Curr Opin Microbiol. 2024 Aug;80:102510. doi: 10.1016/j.mib.2024.102510. Epub 2024 Jul 3. Curr Opin Microbiol. 2024. PMID: 38964276 Review.
-
Predictive Virtual Infection Modeling of Fungal Immune Evasion in Human Whole Blood.Front Immunol. 2018 Mar 21;9:560. doi: 10.3389/fimmu.2018.00560. eCollection 2018. Front Immunol. 2018. PMID: 29619027 Free PMC article.
-
Uniqueness of Candida auris cell wall in morphogenesis, virulence, resistance, and immune evasion.Microbiol Res. 2024 Sep;286:127797. doi: 10.1016/j.micres.2024.127797. Epub 2024 Jun 4. Microbiol Res. 2024. PMID: 38851008 Review.
Cited by
-
"Under Pressure" - How fungi evade, exploit, and modulate cells of the innate immune system.Semin Immunol. 2023 Mar;66:101738. doi: 10.1016/j.smim.2023.101738. Epub 2023 Mar 4. Semin Immunol. 2023. PMID: 36878023 Free PMC article. Review.
-
Tools and techniques to identify, study, and control Candida auris.PLoS Pathog. 2023 Oct 19;19(10):e1011698. doi: 10.1371/journal.ppat.1011698. eCollection 2023 Oct. PLoS Pathog. 2023. PMID: 37856418 Free PMC article. Review.
-
Role of Microbiota in the Skin Colonization of Candida auris.mSphere. 2023 Feb 21;8(1):e0062322. doi: 10.1128/msphere.00623-22. Epub 2023 Jan 25. mSphere. 2023. PMID: 36695588 Free PMC article. Review.
-
Pathobiology of Candida auris infection analyzed by multiplexed imaging and single cell analysis.PLoS One. 2024 Jan 17;19(1):e0293011. doi: 10.1371/journal.pone.0293011. eCollection 2024. PLoS One. 2024. PMID: 38232081 Free PMC article.
-
From environmental adaptation to host survival: Attributes that mediate pathogenicity of Candida auris.Virulence. 2022 Dec;13(1):191-214. doi: 10.1080/21505594.2022.2026037. Virulence. 2022. PMID: 35142597 Free PMC article.
References
-
- Schelenz S, Hagen F, Rhodes JL, Abdolrasouli A, Chowdhary A, Hall A, Ryan L, Shackleton J, Trimlett R, Meis JF, Armstrong-James D, Fisher MC. 2016. First hospital outbreak of the globally emerging Candida auris in a European hospital. Antimicrob Resist Infect Control 5:35. doi:10.1186/s13756-016-0132-5. - DOI - PMC - PubMed
-
- Rudramurthy SM, Chakrabarti A, Paul RA, Sood P, Kaur H, Capoor MR, Kindo AJ, Marak RSK, Arora A, Sardana R, Das S, Chhina D, Patel A, Xess I, Tarai B, Singh P, Ghosh A. 2017. Candida auris candidaemia in Indian ICUs: analysis of risk factors. J Antimicrob Chemother 72:1794–1801. doi:10.1093/jac/dkx034. - DOI - PubMed
Publication types
MeSH terms
Substances
Grants and funding
LinkOut - more resources
Full Text Sources
Molecular Biology Databases
Miscellaneous